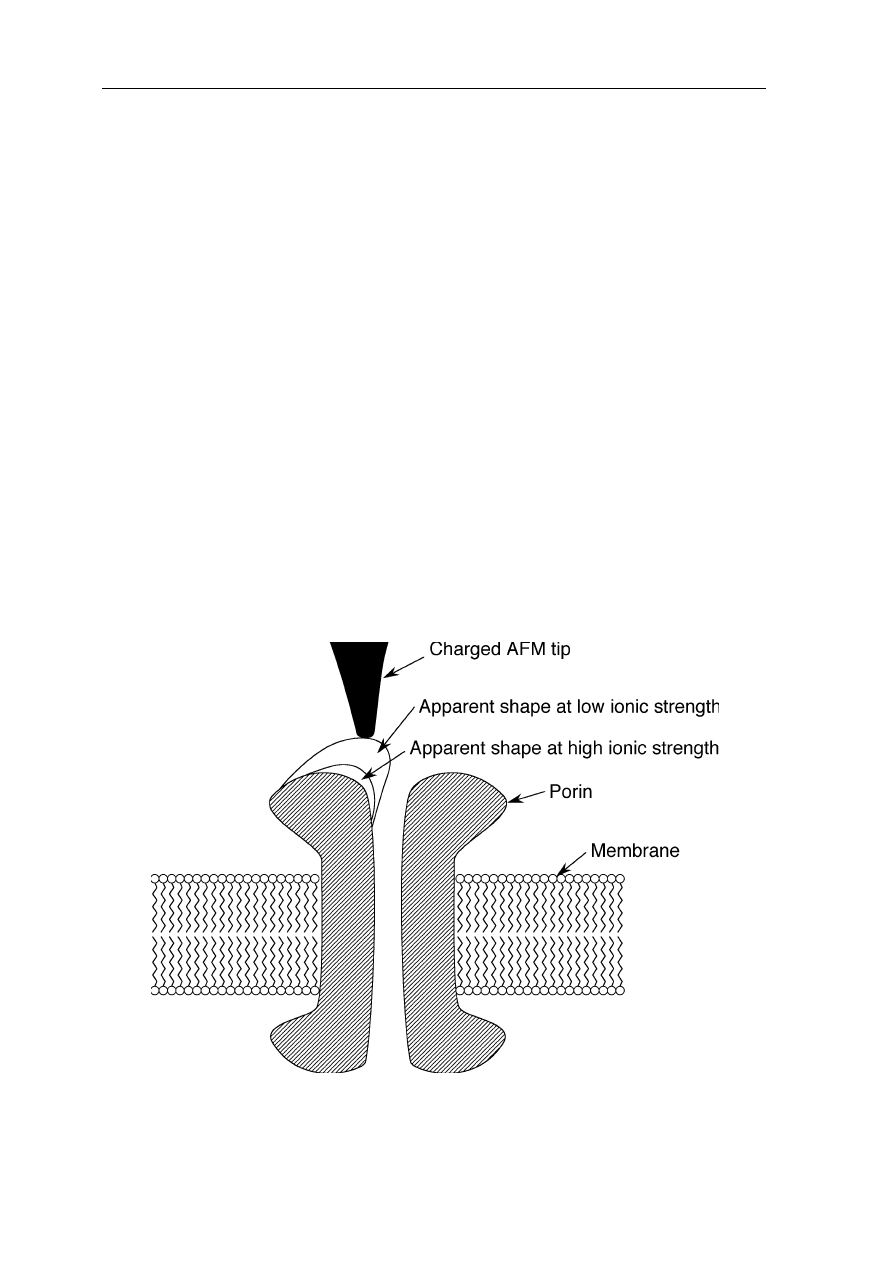
7.1 Atomic force microscope (AFM) 131
Morris, 2002). AFM technology was used to map out the electrostatic potential of
the transmembrane channel OmpF porin (Fig. 7.16; Philippsen et al., 2002).
AFMs gave crucial topological information of blood cell adhesion on different
sensor materials (Hildebrand et al., 2001). Ac-GWWL(AL)nWWA-Etn peptides
induce the formation of extremely ordered domains in some biologically relevant
membranes (Rinia et al., 2002). The heads of bacteriophage
Φ
KZ and T4 have
different compressibilities (Matsko et al., 2001). Atomic force microscopy
resolved fusion pores in the apical plasma membrane in live pancreatic cells (Cho
et al., 2002) and visualized the growth of Alzheimer's
β
-amyloid-like fibrils
(Goldsbury et al., 2001). Cardiac muscle and skeletal muscle exhibit different
viscous and elastic properties as determined by atomic force microscopy. Cardiac
cells are stiffer (elastic modulus = 100
±
11 kPa) than skeletal muscle cells (elastic
modulus = 25
±
4 kPa; see Mathur et al., 2001). Atomic force microscopy
allowed to visualize the structure of biomolecules, e.g., the native chaperone
complex from
Sulfolobus solfataricus
, in solution under physiological conditions
providing a nanometer resolution topographic image of the sample (Valle et al.,
2001). It is also an excellent technique to study the initial events of mutual cell
adhesion (Razatos, 2001). An AFM image of a monomolecular film of bovine
serum albumin shows individual monomers and dimers (Fig. 7.17; Gunning et al.,
1996; Morris et al., 1999).
Fig. 7.16
Imaging the electrostatic potential of the transmembrane channel OmpF porin
(Philippsen et al., 2002). Different apparent shapes of the porin are observed at different
ionic strengths. These differences reflect changes of the electrostatic potential which is
experienced by the charged tip of the AFM