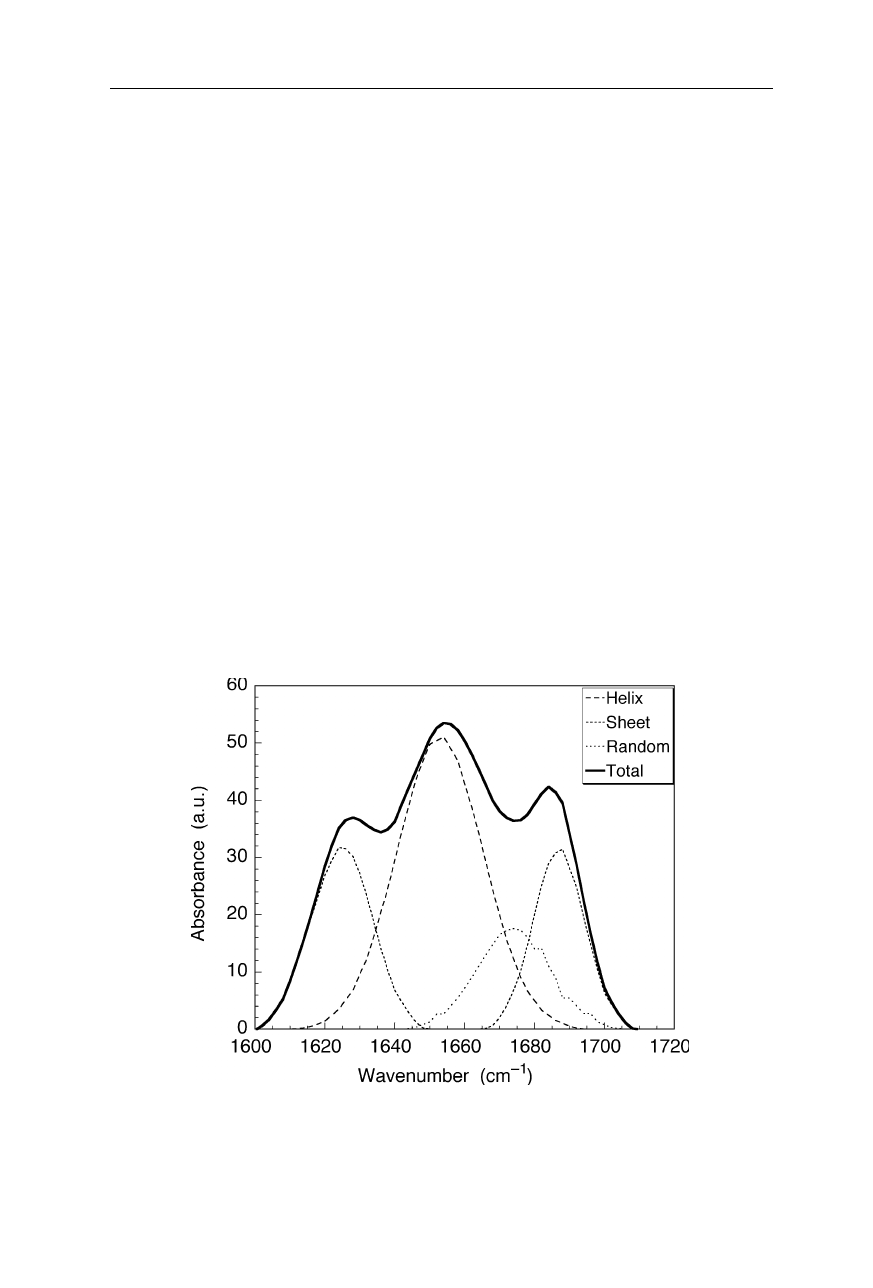
104 5 Protein infrared spectroscopy
vibrations of the phosphate calcium ATPase complex were detected at a
background of 50,000 protein vibrations in an isotope exchange experiment
(Barth, 2002).
Time-resolved step-scan FTIR spectroscopy enables the monitoring of confor-
mational changes of proteins in the microsecond time scale (Bailey et al., 2002).
FTIR spectroscopy allowed to map out the nucleotide binding site of calcium
ATPase (Liu and Barth, 2002). IR and FTIR spectroscopy are two of the only few
methods suitable to monitor conformational changes of proteins under high
pressure (Fig. 5.16; Dzwolak et al., 2002). FTIR spectroscopy on bacterio-
rhodopsin revealed a pre-melting conformational transition at 80
o
C (Heyes et al.,
2002). FTIR is also suitable to investigate the structure and hydration shell of
protein molecules in organic solvents (Fig. 5.17; Costantino et al., 1995). Further,
IR and FTIR spectroscopy was used for the characterization of irradiated starches
(Kizil et al., 2002), and the determination of dihedral angles of tripeptides
(Schweitzer-Stenner, 2002). Molecular changes of preclinical scrapie can be
detected by IR spectroscopy (Kneipp et al., 2002). FTIR spectroscopy can serve
as an optical nose for predicting odor sensation (van Kempen et al., 2002) and for
chemical analysis of drinks (Coimbra et al., 2002; Duarte et al., 2002).
FTIR microscopy at a spatial resolution of 18
µ
m resolved single cells (Lasch
et al., 2002). IR spectroscopy is also a tool for discrimination between different
strains or types of cells (Gaigneaux et al., 2002).
Fig. 5.18
Decomposition of a FTIR spectrum into three components corresponding to
helical structure, sheets and non-regular structure, respectively. Percentages of structure
content and structural changes, e.g., due to protein denaturation, are quantifiable