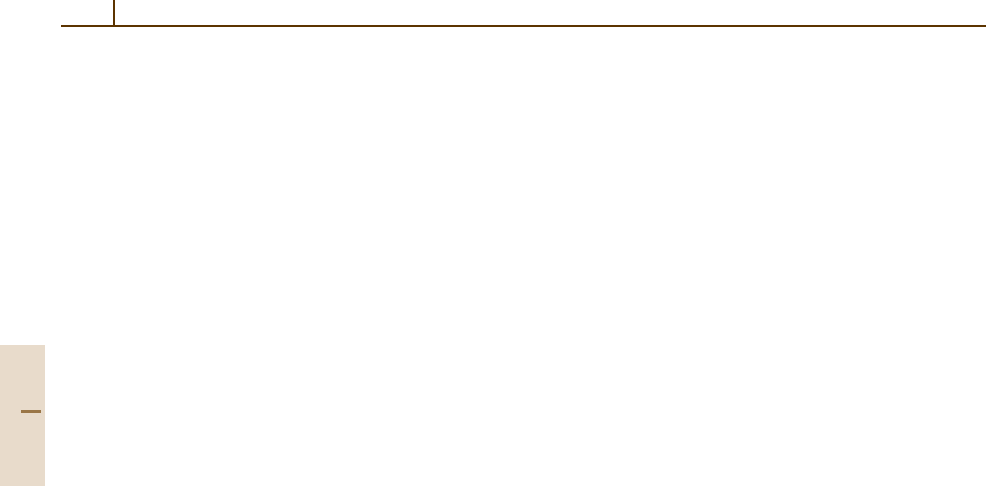
1060 Part 5 Special Structures
bit. The readout of the stored information in the se-
lected bit is done by applying a bias between the
lines that cross it and measuring the current that flows
through it.
For the successful realization of MRAM devices us-
ing MTJs, two very critical components – the tunnel
barrier and the free layer – have to be optimized. The
tunnel barrier needs to show large-scale uniformity over
the wafer, in addition to the more or less obvious pre-
requisites of being pinhole-free and extremely smooth.
This is because the tunnel resistance depends exponen-
tially on the barrier thickness and the absolute value of
the MTJ resistance is compared with a reference cell
during the read operation. As far as the free layer is
concerned, its thickness is directly related to the value
of the magnetic field or current required for switching
a bit. A free layer less than 5 nm thick is desirable, since
it results in a low switching field and, as a consequence,
low power consumption.
A number of difficulties have to be resolved to create
successful devices. These include efficient spin injection
into semiconductors and heterostructures, and a search
for new spin-polarized materials. Other effects poten-
tially important for spintronic devices include optical
and electrical manipulation of ferromagnetism, current-
induced switching and precessing of magnetization, and
the possibility of a long coherence time for optically ex-
cited spins in semiconductors. For a good overview of
the issues in spin electronics, including the prospects for
spintronic quantum devices, see [3.118].
5.3.4.2 Ultrahigh-Density Storage Media
in Hard Disk Drives
The Limits of the Present Technology
In the current storage technology, which uses continu-
ous polycrystalline thin-film media, the signal-to-noise
ratio (SNR) of the medium is directly related to the
number of grains per bit. At storage densities on the
order of 80 Gb/in
2
, the grain size of the CoCrPtX al-
loys (X = Ta, P, etc.) used in the current media is on the
order of 8 nm, the number of grains per bit is about 100,
and the bit size is on the order of 250 nm×30 nm. A fur-
ther reduction in the number of grains per bit below 100
would lead to a strong decrease in the SNR. To continue
decreasing the bit cell size in continuous media (while
keeping the SNR constant), it is necessary to reduce the
grain size further. However, there is a physical limit to
how small the grain size can be before thermal varia-
tions can affect the stability of the magnetization. This
is known as the superparamagnetic limit. In CoCrPt al-
loys, the minimum grain size for which the criterion for
magnetic stability can be satisfied is about 8 nm, which
is the grain size used in today’s media. Further reduc-
ing the grain size requires the use of other materials
as FePt, SmCo, and FeNdB alloys. However, this im-
plies that larger write fields will be needed to switch
the magnetization of the grains. This would necessi-
tate the development of new materials which are not yet
known. In the short term, the use of antiferromagnetic-
ally coupled media has allowed the superparamagnetic
limit to be extended in combination with GMR read
heads [3.119].
The Promise of Perpendicular Media
It is foreseen that within two or three years, magnetic
recording will switch to perpendicular discontinuous
media for several reasons. In perpendicular media, as
opposed to longitudinal granular media, the smaller the
bit size, the smaller the effect of the demagnetizing field
on each bit is. In addition, the efficiency of the writer can
be greatly improved, and higher-anisotropy materials
can be used. Both effects contribute to a better thermal
stability at small size grain size as compared with longi-
tudinal media. This should help us to reach densities of
the order of 200–400 Gb/in
2
. However, to progress be-
yond these densities, a totally different approach will be
required, which may consist of using patterned media,
i. e. media made of arrays of nanometer-scale individual
dots, in which each dot carries a bit of information. The
significant advantage of this type of medium is that the
shape and edge of each bit are not determined by the
grain size as in continuous media, but are determined
by the patterning process used to make the dots. Con-
sequently, each dot can be made of a single magnetic
domain (1 grain per bit instead of the 100 grains per
bit in a continuous medium). The volume of a grain
can therefore be much larger in a patterned medium so
that the superparamagnetic limit is no longer a prob-
lem, at least up to several terabits per square inch. Of
course, the use of patterned media raises other ques-
tions such as fabrication at low cost, tracking, and
reproducibility.
Various techniques have been developed to pre-
pare arrays of submicrometer magnetic dots: optical,
electron-beam, or X-ray lithography followed by
etching of the magnetic material; patterning using
a focused beam [3.120]; and self-organization of nano-
particles [3.121]. So far, however, none of these
techniques seems to be able to combine the criteria of
low cost, and rapid patterning of large areas (several
square inches) down to 25 nm pitch.
Part 5 3.4