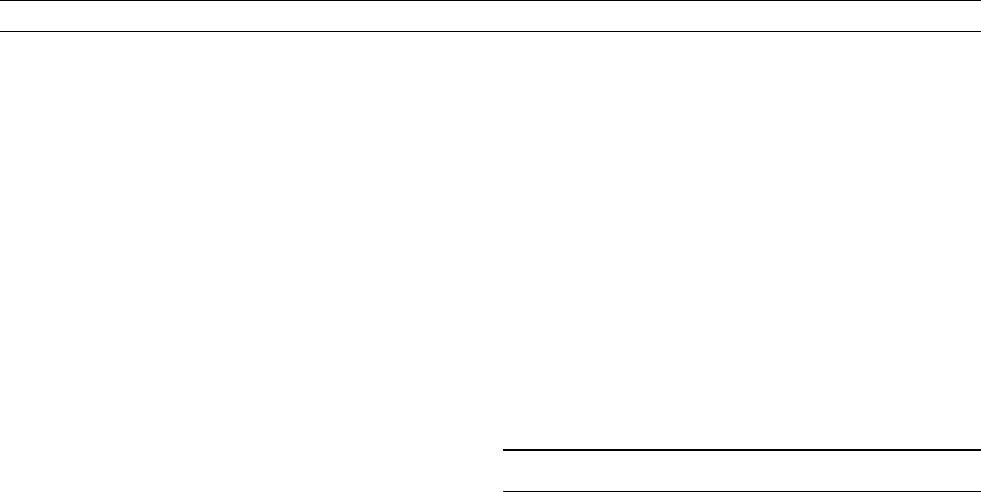
explain the strong seismic reflectivity in the middle crust. An unex-
pected result of the MT image is the lack of correlation with the
down-going slab (NAZCA reflector) or the Quebrada Blanca Bright
Spot (QBBS). If the high reflectivity of the QBBS would be caused
by large volumes of entrapped fluids ascending through the crust,
however, then this should lead to a significant increase in the electrical
conductivity. Obviously, the MT data contradict this interpretation, and
it is also possible that the reflector is due to some strong structural con-
trast. The down-going slab and the domains immediately above it
could become electrically conductive (i) because of water-rich sedi-
ments being transported in the subduction system or (ii) by release
of fluids in the blueschist-eclogite transition zone in 80–100 km
depth. Modeling studies show that such a conductor is compatible with
the data (see Schwalenberg et al., 2002; and H. Brasse, personal com-
munication) but it is not required to fit the data. Hence, if an electri-
cally conductive slab exists, it is obscured by the overwhelming
lateral influence of the high conductivity zones associated with the
Pacific Ocean in the west and the Altiplano in the east.
Oliver Ritter
Bibliography
Bedrosian, P., Unsworth, M., Egbert, G., and Thurber, C., 2004. Geo-
physical images of the creeping segment of the San Andreas Fault:
implications for the role of crustal fluids in the earthquake process.
Tectonophysics, 385: 137–158.
Brasse, H., Lezaeta, P., Schwalenberg, K., Soyer, W., and Haak, V.,
2002. The Bolivian Altiplano conductivity anomaly. Journal of
Geophysical Research, 107(5): 10.1029/2001JB000391.
Jödicke, H., Kruhl, J.H., Ballhaus, C., Giese, P., and Untiedt, J., 2004.
Syngenetic, thin graphite-rich horizons in lower crustal rocks from
the Serre San Bruno, Calabria (Italy), and implications for the
nature of high-conducting deep crustal layers. Physics of the Earth
and Planetary Interiors, 141:37–58.
Nover, G., Stoll, J.B., and Gönna, J., 2005. Promotion of graphite for-
mation by tectonic stress—a laboratory experiment. Geophysical
Journal International, 160: 1059–1067.
Pous, J., Munoz, G., Heise, W., Melgarejo, J.C., and Quesada, C.,
2004. Electromagnetic imaging of Variscan crustal structures in
SW Iberia: the role of interconnected graphite. Earth and Plane-
tary Science Letters, 217: 435–450.
Ritter, O., Ryberg, T., Weckmann, U., Hoffmann-Rothe, A., Abueladas,
A., Garfunkel, Z., and DESERT Research group, 2003. Geophysical
images of the Dead Sea transform in Jordan reveal an impermeable
barrier for fluid flow. Geophysical Research Letters, 30(14): 1741,
doi:10.1029/2003GL017541.
Ritter, O., Hoffmann-Rothe, A., Bedrosian, P.A., Weckmann, U., and
Haak, V., 2005. Electrical conductivity images of active and fossil
fault zones. In Bruhn, D., and Burlini, L. (eds.), High-Strain Zones:
Structure and Physical Properties, Vol. 245. Geological Society of
London Special Publications, pp. 165–186.
Schilling, F.R., and Partzsch, G., 2001. Quantifying partial melt frac-
tion in the crust beneath the central Andes and the Tibetan Plateau.
Physics and Chemistry of the Earth, 26: 239–246.
Schwalenberg, K., Rath, V., and Haak, V., 2002. Sensitivity studies
applied to a two-dimensional resistivity model from the Central
Andes. Geophysical Journal International, 150(3): 673–686.
Unsworth, M.J., Malin, P.E., Egbert, G.D., and Booker, J.R., 1997.
Internal structure of the San Andreas fault at Parkfield, California.
Geology, 25(4): 359–362.
Weckmann, U., Ritter, O., and Haak, V., 2003. A magnetotelluric
study of the Damara Belt in Namibia 2. MT phases over 90
reveal
the internal structure of the Waterberg Fault/Omaruru Lineament.
Physics of the Earth and Planetary Interiors, 138:91–112, doi:
10.1016/S0031-9201(03)00079-1.
Cross-references
Anisotropy, Electrical
Electromagnetic Induction (EM)
EM Modeling, Forward
EM Modeling, Inverse
EM, Industrial Uses
EM, Land Uses
EM, Marine Controlled Source
EM, Tectonic Interpretations
Geomagnetic Deep Sounding
Induction Arrows
Induction from Satellite Data
Magnetotellurics
Natural Sources for EM Induction Studies
Robust Electromagnetic Transfer Functions Estimates
Transfer Functions
Transient EM Induction
EM, TECTONIC INTERPRETATIONS
Studies of electromagnetic induction in the Earth are used to construct
models of the variation of electrical conductivity in 1, 2, or 3 dimen-
sions. Although the goal of some studies is the delineation of mineral
or geothermal resources, the aim of many such investigations of con-
ductivity structure is an improved understanding of tectonic processes.
On a local scale these may include studies of active fault zones or vol-
canoes, while regional-scale studies include investigations of subduc-
tion zones, orogenic belts (regions of mountain building), and even,
using seafloor measurements, spreading ocean ridges and mantle hot
spots.
Electromagnetic (EM) methods can be used to study active tectonic
processes primarily because fluids (molten rock or aqueous solutions)
are typically either formed or released during these processes. In com-
parison to background values of the electrical resistivity of crustal
rocks (100s–1000s Om), both partial melts and aqueous fluids are
highly conductive and can have the effect of lowering the bulk resis-
tivity of host rocks, to the extent that they form ideal targets for detec-
tion using EM techniques such as magnetotelluric (MT) sounding. The
degree to which the bulk resistivity of a rock is reduced by the pre-
sence of fluids depends primarily on two factors. The first of these is
the resistivity of the fluid itself, but more important is the degree to
which the fluid forms an interconnected network and, thus, a continu-
ous electrically conductive path through the host rock. For example,
simple numerical calculations of the bulk resistivity of a two-phase
system (Figure E29) shows that isolated pockets of fluid (e.g., molten
rock) with a resistivity of 0.1–0.25 Om have little effect on the bulk
resistivity of a solid host rock with a resistivity of 1000 Om even if
the melt forms 10% by volume. In contrast, a connected melt of as
a little as 1% by volume, distributed through the host rock, reduces
the bulk resistivity by a factor of nearly 100. The minimum amount
of melt or aqueous fluid that is necessary for the fluid to be connected
is surprisingly small and possibly less than 1% (Minarik and Watson,
1995).
An excellent example of the manner in which the presence of both
partial melt and aqueous fluids facilitates the use of EM methods to
investigate tectonic processes is presented by the results of the electro-
magnetic sounding of the lithosphere and asthenosphere beneath
(EMSLAB) study of the Juan de Fuca subduction zone off the Pacific
Coast of North America (Wannamaker et al., 1989). The two-dimensional
model of electrical resistivity (Figure E30), derived from MT data
along a transect of over 300 km in length, shows several regions of
anomalously low resistivity. In particular, a thin region of low resis-
tivity dipping to the east below the North American continent is inter-
preted as being due to the presence of residual oceanic sediments on
EM, TECTONIC INTERPRETATIONS 245