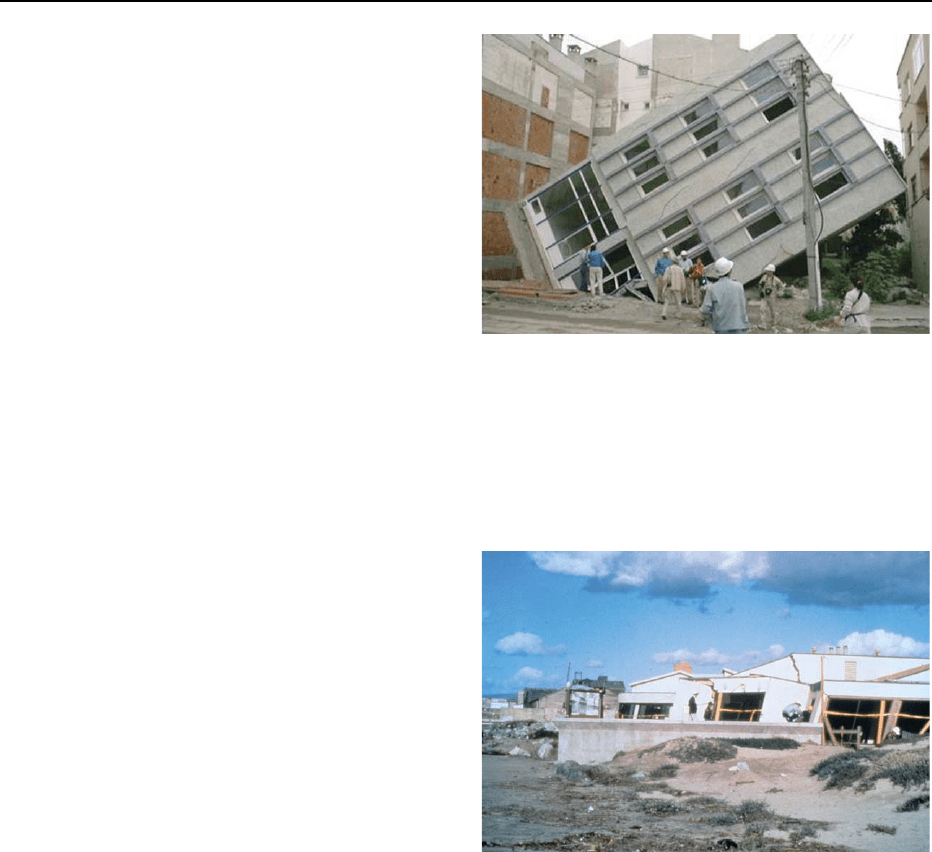
can reduce the shear strength. Field data, however,
are implicitly likely to include such phenomena. Con-
sequently, the use of the empirical correlations to
estimate postliquefaction shear strengths is recom-
mended.
Consequences of Earthquake-
Induced Liquefaction
The importance of liquefaction from an engineering
aspect is damage to the built environment, as repre-
sented by the following examples:
.
Settlements, which can be of the order of 5% of the
thickness of the liquefied soil, causing uniform or
differential settlement of foundations, pipelines, or
transportation routes.
.
Damage to piled foundations as result of loss of
support and flow of soil around piles that extend
into or through the liquefied soil layer(s).
.
Loss of bearing support to shallow or piled foun-
dations causing settlement or tilt.
.
Lateral spreads damaging building foundations,
bridge piers, highways and railways, river banks,
or embankment dams or pipelines.
.
Lateral flow failures.
.
Embankment failures due to liquefaction of under-
lying material or embankment fill.
.
Service interruption to buildings due to connection
damage caused by foundation displacements.
.
Increased lateral pressures against retaining struc-
tures such as quay walls.
.
Induced hazards, such as fire caused by gas pipeline
rupture, spread of fire due to interrupted water
supply, or floods caused by dam failure.
The severity of the damage is dependent on the
strength and duration of the earthquake ground
shaking, the thickness of the liquefied layer, the ma-
terial properties of the liquefied soil, and the proxim-
ity of free faces such as slopes or retaining structures,
as well as other variables related to the structures. The
response of a building to liquefaction-induced vertical
or lateral permanent ground deformation depends
to a large extent on the building’s foundations
(Figures 13–15). Bridge embankments are vulnerable
to lateral spreading due to the combination of suscep-
tible river channel deposits and sloping or free faces.
Bridge piers in liquefied ground can rotate, twist, or
displace laterally. In extreme cases, when liquefaction
is combined with poor design in the form of insuffi-
cient bearing width of the bridge deck, excessive
movement can cause loss of support to the bridge
deck and hence collapse (Figure 15). Damage to
roadways or railways due to liquefaction most
commonly comprises settlement or lateral spreading
of embankments, highway fills, or natural soil, which
causes cracking and uneven surfaces. Such damage is
relatively easy and inexpensive to repair but, nonethe-
less, the disruption and the indirect losses must be
considered.
The performance of a region’s lifelines networks,
including water, electricity, and gas distribution
systems, is an essential factor in the immediate emer-
gency response and the subsequent recovery from the
earthquake impact. Pipeline damage can be induced
both by transient ground oscillations and by vertical
Figure 13 Tilted apartment block in Adapazari, Turkey, due to
extensive liquefaction in the 1999 magnitude 7.4 Kocaeli earth-
quake. This building had thick, continuous, shallow foundations,
which caused it to rotate as a rigid body. There is very little
damage to load-bearing elements, walls, or ceilings; nonethe-
less, the building would have been demolished. Photograph by
Beyza Taskin.
Figure 14 Damage to the Marine Laboratory at Moss Landing,
Monterey Bay, California, during the 1989 magnitude 6.9 Loma
Prieta earthquake. Lateral spreading caused the building pad
foundations to spread apart. The laboratory was subsequently
demolished. Photograph by Leslie F. Harder, Jr.
532 ENGINEERING GEOLOGY/Liquefaction