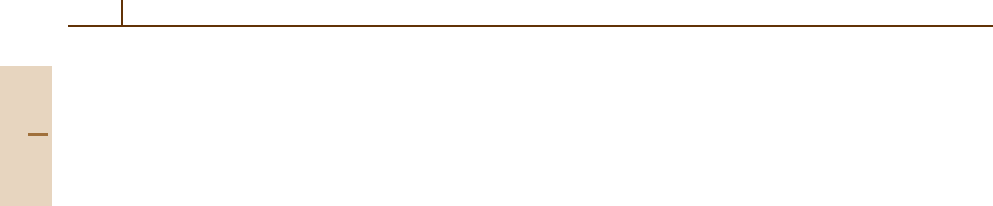
1348 Part G Aplications
as a natural companion to UPS. IPES utilizes low energy
electrons (5–50 eV) incident on a surface. Transitions
from a high-lying initial electron state in the contin-
uum to a lower unoccupied state cause a UV photon
to be emitted. By detecting the emitted photon inten-
sity as a function of energy, the joint density of states is
measured [89.7]. IPES is used to study the unoccupied
portion of surface and bulk bandstructure, particularly
the region between the Fermi level and the vacuum level,
which is difficult to access by other means. This allows
the study of unoccupied states of adsorbed molecules
(e.g., antibonding molecular levels) as well as intrinsic
surface states such as the Rydberg-like states of electrons
trapped in the image potential at the surface [89.7].
89.3.3 X-Ray Photoelectron
Spectroscopy (XPS)
X-ray photoelectron spectroscopy allows the study of
the energy of atomic core levels via the Einstein photo-
electric equation T
e
= hν − E
b
,whereT
e
is the kinetic
energy of the photoemitted electron and E
b
is the
binding energy of the core level. Atoms bound in dif-
ferent chemical environments (e.g., at particular sites
on a surface) experience different chemical shifts, and
so are measured at slightly different binding energies.
This sensitivity to chemical environment allows XPS to
characterize the different types of binding sites for sim-
ilar atoms, and measure their abundance by measuring
the relative intensities of XPS emission from different
species.
Shifts in T
e
arise from two sources: intra-atomic
and inter-atomic relaxation shifts. The intra-atomic re-
laxation E
a
is due to screening of the core hole by other
electrons in the emitter atom. The inter-atomic relax-
ation E
r
is important in solids (particularly for metals)
and is due to screening of the core hole by the dielectric
response of the surrounding medium. These relaxation
shifts (on the order of a few eV) tend to increase the ki-
netic energy of the emitted photoelectron, so the kinetic
energy in the adiabatic limit is
T
e
= hν − E
b
+ E
a
+ E
r
. (89.7)
Since photoemission is a rapid process, nonadiabatic
processes lead to shakeup and shakeoff features in which
other electrons are excited to higher energy levels, caus-
ing the photoelectron to have lower kinetic energy [89.5]
(see Sect. 62.4.4). It is also possible to excite discrete
excitations of the solid, such as plasmons and a contin-
uum of low energy electron-hole excitations, causing the
XPS peak to have an asymmetric lineshape. The over-
all XPS distributions of T
e
thus contain contributions
from the adiabatic channel, and lower energy photoelec-
trons in a series of discrete peaks or a continuum from
nonadiabatic processes.
The chemical abundance of a species can be deter-
mined from the sum rule that the total XPS cross section
is proportional to the sum of the adiabatic peak and
all the shake-up and shake-off components. However it
is usually only convenient to measure the intensity of
the adiabatic peak. If comparisons are made between
chemical species in different chemical environments,
the shake-up and shake-off intensities may differ. Hence
the adiabatic channel intensities might not reflect the
true abundance. Very often this problem can be mini-
mized by careful calibration, and chemical analyses can
be made to an accuracy of a few percent.
The surface sensitivity of XPS is due to the short in-
elastic MFP for the photoelectrons, which can be made
to have energies in the range 10–1000 eV by appropriate
choice of the X-ray wavelength. The XPS signal is pro-
portional to exp[−z/(λ cos θ)],wherez is the depth of
the emitter, λ is the inelastic mean free path of the pho-
toelectron and θ is the angle measured from the surface
normal.
The popularity of XPS as a surface analysis tech-
nique is due to the availability of convenient and
sufficiently intense monochromatic X-rays from lab
sources (usually Al and Mg K
α
X-ray lines at 1486.6
and 1253.6 eV). The increasing availability of con-
tinuously tunable X-rays from synchrotron radiation
sources allows improved measurements due to the abil-
ity to tune the X-rays to slightly above threshold,
where the XPS cross-sections are maximized, the in-
elastic MFP is shorter (hence more surface specific)
and electron monochromators can operate with higher
resolution [89.5].
As an example of the chemical sensitivity of XPS,
photoelectron spectra of the 4f
7/2
core levels of W(111)
and Ta(111) are shown in Fig. 89.3. The spectra show
discrete peaks from both bulk and surface atoms. The
binding energies of the surface atoms are affected by the
adsorption of hydrogen.
XPS can also be used to give local structural in-
formation due to elastic scattering of the photoemitted
electron in the region near the emitter [89.5]. One form
of this is the use of forward scattering from a buried
emitter. A high energy XPS photoelectron is focused in
the direction of nearby atoms due to a lower effective
potential close to the atomic core. Angular scanning of
the XPS detector will then detect a more intense signal
along the bond axis. This method has proved useful in
Part G 89.3