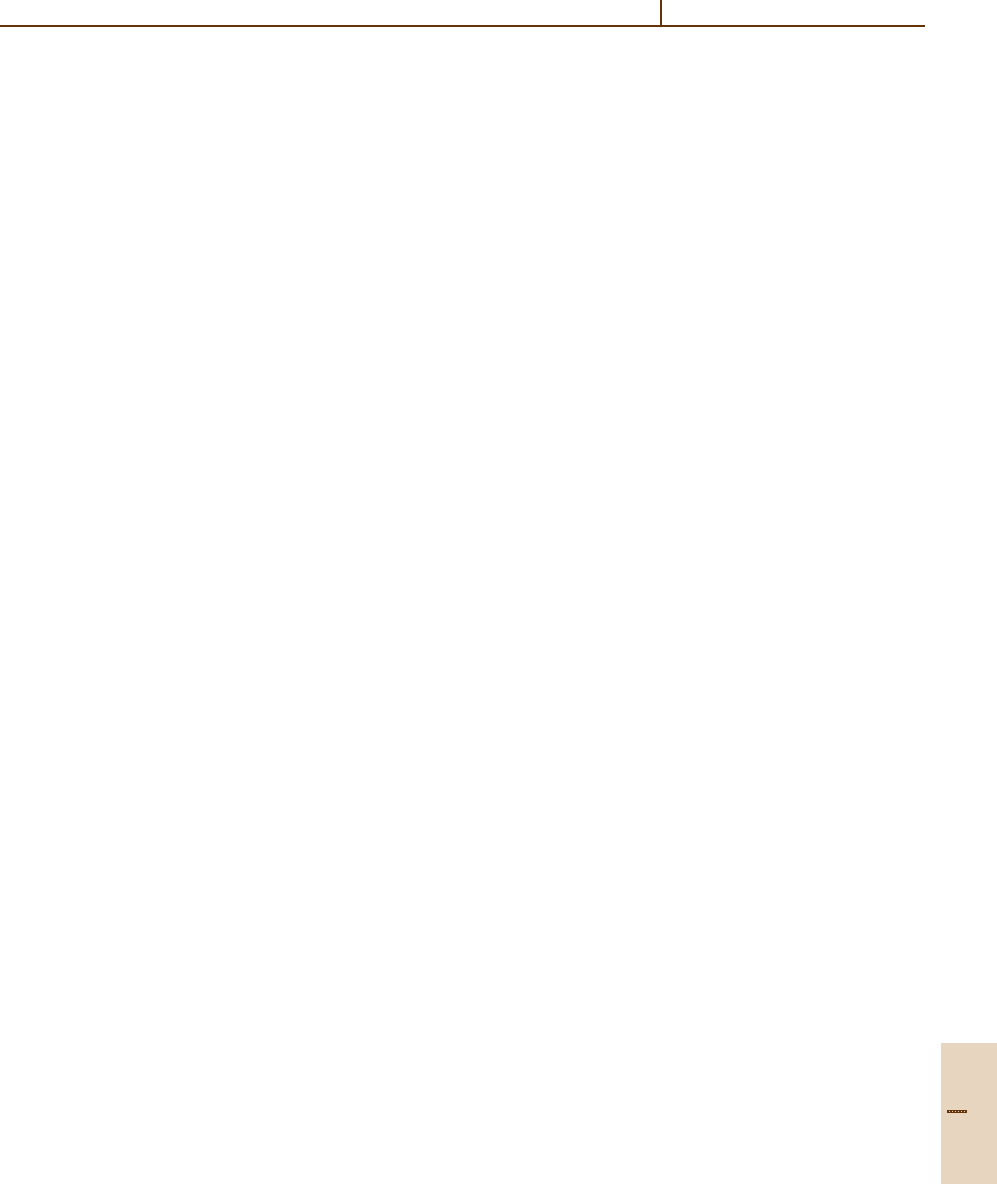
Applications to Combustion 88.2 Laser Combustion Diagnostics 1341
than in the ground state. Rotational transfer in OH has
been shown to have some definite propensities, including
parity conservation for some collisions with He that is
reproduced in quantum scattering calculations.
Such a wealth of detail is not yet available for other
free radical combustion intermediates, although they are
beginning to be investigated. Of particular current inter-
est is NO and the major precursor to its formation, CH.
The details of quenching by a variety of colliders are
probably known well enough that quantum yields for
OH, NO, and CH may be calculated reasonably accu-
rately, given the local temperature and major species
flame composition at the point of measurement. There
are enough data to compute quenching rates to within
about 30–50% for many flames; this is partly because
H
2
O, when present, accounts for the majority of the
quenching for all three radicals. When the local environ-
ment (especially T) is not known, the situation is worse.
This would arise for an instantaneous two-dimensional
image of a radical in a flame. Even so, the net quenching
rate for OH rarely varies more than threefold through
the entire flame, so useful semiquantitative information
can still be obtained.
If absolute LIF measurements are desired, some
means of calibration is necessary. Stable compounds
(NO, CO) can simply be introduced into the flames or
corresponding cold flows. Free radicals pose a greater
problem. OH is often present in sufficient quantity
that absorption measurements in a stable, laminar one-
dimensional flame can be performed, but this is generally
not possible for other species. The recently introduced
technique of cavity ring down spectroscopy may alter
this situation [88.10]. Burnt gases, in thermal equilib-
rium at a measured T, furnish a reliable source of known
concentrations of OH, H, and O but generally not other
radicals (which are consumed earlier in the flame).
Two-photon excitation is used for detection of
atomic species and the CO molecule. The high laser flux
needed for two-photon absorption, together with the uv
wavelengths required for the pertinent transitions, of-
ten leads to problems with photochemical interferences.
Sometimes these produce the same species that is be-
ing measured, e.g., dissociation of vibrationally excited
O
2
at the wavelength of atomic oxygen detection, with
subsequent excitation of the spurious O atom. In other
cases, especially in complex hydrocarbon fuels, a par-
ent compound or partially oxidized fragment may be
excited directly or photolyzed to yield an emitting prod-
uct. Excitation scans and measurements as a function
of laser power are needed to discern and avoid these
interferences.
88.2.3 Degenerate Four-Wave Mixing
DFWM is a nonlinear process like CARS; but like LIF,
it operates on real transitions. It combines some of the
attributes of both methods: production of a coherent
signal beam that can be spatially filtered to discrim-
inate against background, and high sensitivity so that
trace radical species may be detected [88.11]. It depends
on χ
(3)
,asdoesCARS, and can be used in a broadband
mode. Like LIF, it can produce two-dimensional im-
ages, with the proper laser sheet arrangement. DFWM
adds some of its own advantages, especially a Doppler-
free nature enhancing the spectral resolution and thus
the molecular selectivity. Three laser beams are used
to generate the DFWM signal, all the same frequency,
and tuned to an absorption transition of the species of
interest. Two pump beams create an interference pat-
tern in the medium, and a probe beam scatters off the
resulting grating to form the signal. The interference pat-
tern may be in the ground/excited state populations or
may exploit polarization phenomena. Rapid quenching
of the excited state may produce a thermal grating in
the medium; the probe beam can then scatter from the
resulting variation in the index of refraction. DFWM de-
pends only on absorption and may be used for sensitive
detection in a nonfluorescing or poorly fluorescing case
(e.g., a predissociative state or ir vibrational transition).
The interpretation of DFWM signals to obtain
molecular concentrations or temperatures requires
knowledge of the underlying physical phenomena. Be-
cause they are governed by χ
(3)
,theDFWM amplitudes
interfere, and an understanding of molecular collisions,
motion, and the effects of the laser power are needed
for accurate modeling of DFWM spectra. Collisional
effects are of particular interest due to the Doppler-
free character of the technique. Signal intensities can
be dramatically influenced by line broadening due to
quenching, energy transfer, and dephasing collisions.
The magnitude of the influence depends sensitively on
the degree of optical saturation. A cohesive picture of
these effects is currently being sought through a combi-
nation of measurement and theory. Furthermore, nearly
all current theoretical treatments assume single-mode
lasers; however, real lasers contain intensity and fre-
quency fluctuations within each laser pulse. The effects
of these fluctuations on the nonlinear wave-mixing pro-
cess must be accounted for in a proper description
of DFWM. Furthermore, because collisions affect the
signal only during the laser pulse, rapid (ps) measure-
ments may provide excellent sensitivity under very high
P conditions.
Part G 88.2