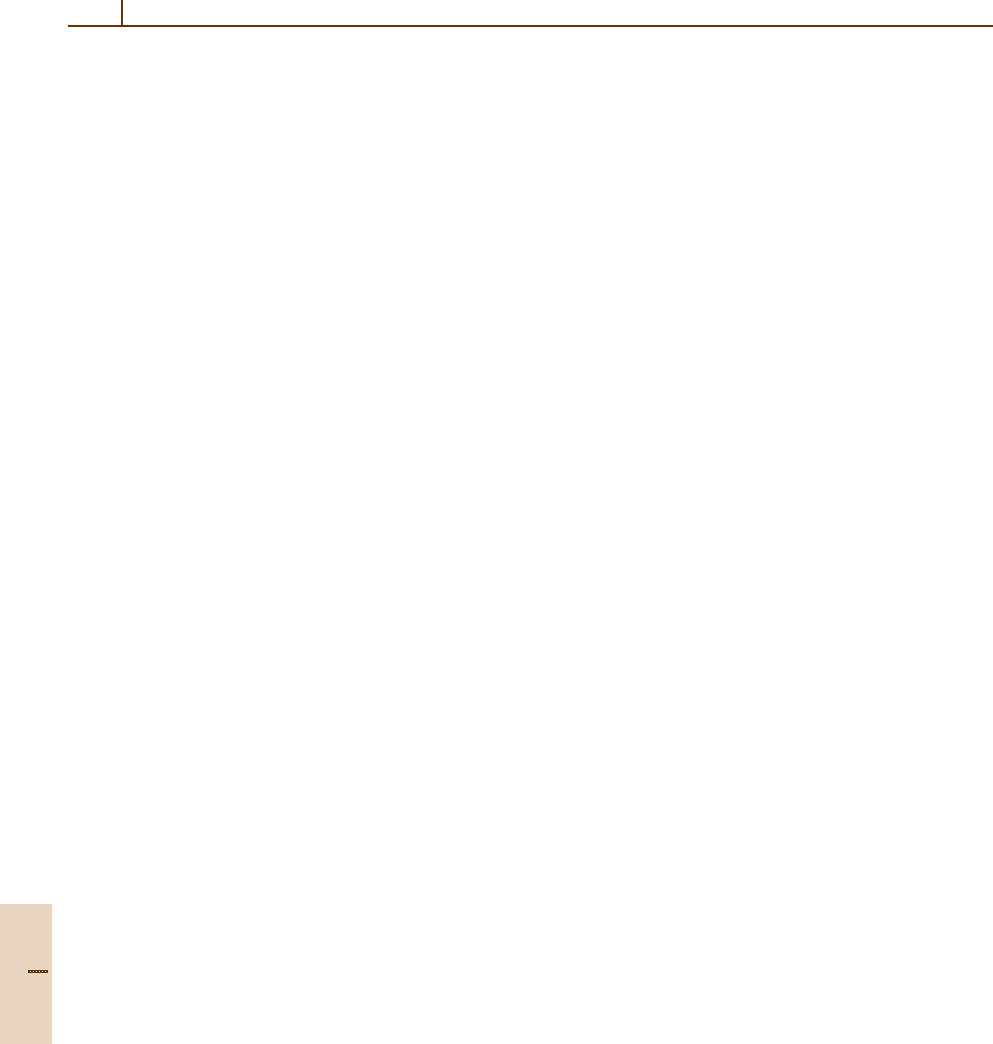
1332 Part G Applications
the rf excitation frequency. The anisotropy and selec-
tivity of the etching processes are determined by the
ion energy and its directionality, the etching radical,
and the substrate materials. Above energies of several
hundred volts, ion bombardment of the substrate causes
sputtering, which is relatively unselective. Pure chem-
ical etching by radicals alone may be selective, but it
is relatively isotropic. The aim in discharge enhanced
processing is to obtain plasma conditions that generate
selected radicals with high efficiency, concurrent with
bias voltages that provide highly directional ions to pre-
pare the substrate for etching. The combination of these
processes gives etch rates that are typically an order of
magnitude higher than the sum of the individual pro-
cesses. The microstructures that can be fabricated using
processing are critical to all of high density integrated
circuit manufacturing. The achievements are illustrated
by 0.2 µm wide trenches in silicon that are 4 microns
deep with vertical sides [87.64].
On the other hand, the electrons form an energy-
modulated fast electron beam that is injected into the
plasma region. These fast electrons maintain much of
the ionization in a symmetrically excited, equal elec-
trode area reactor. In principle, it is possible to develop
a complete self-consistent model of the rf discharge,
consisting of the Boltzmann equation for the energy dis-
tribution function of the electrons, positive ions, and
negative ions coupled to the Poisson’s equation for
the electric field. Simpler models based on moments
of the Boltzmann equation have been developed for
higher pressures [87.65] (where a fluid approximation
is more appropriate) and particle-in-cell Monte-Carlo
simulations for low pressures [87.64, 66]. The above
studies have led to the identification of four prin-
cipal mechanisms affecting energy deposition. These
involve:
1. the impedance of the bulk plasma;
2. the energy deposition by fast electrons created by
secondary emission due to ion bombardment of the
electrodes;
3. collisionless absorption due to the asymmetrical
sheath boundary-plasma electron interaction; and
4. wave-riding, or collisional sheath interactions caus-
ing electron heating in the sheath modulated electric
field. The electron is regarded as surfing on the
expanding sheath field.
Processes (3) and (4) are related, with the difference
being that in (4) electron collisions occur during the
sheath expansion. There is also an additional interaction
due to the changes in the complex impedance (capacitive
sheaths and resistive bulk plasma) of the discharge and
the consequent changes in the power transferred from
the rf generator. The mechanism of collisionless heating
is an active research area [87.67]. It is proposed that
low pressure rf plasmas can be maintained mainly by
collisionless heating in the rf modulated sheaths, and
that electron inertia plays a dominant role.
Other less general plasma modes occur due to res-
onance when the electric field and period are such that
one electron transit requires one half period of the rf
cycle. Under these conditions at very low pressures,
when λ
mfp
is larger than the gap spacing, and secondary
electron emission can occur, one has the multipactor
discharge mode. Otherwise, when λ
mfp
corresponds
approximately to small integral fractions of the gap
spacing, plasmoid modes are excited.
Dielectric Barrier Discharge
In many applications, such as ozone generation (for wa-
ter treatment), distributed uv sources, and the creation
of radicals for surface treatments, there are economic
incentives to operate at high pressures, especially at
atmospheric pressure. The dielectric barrier discharge
(DBD, also called the silent discharge in earlier liter-
ature) is a high voltage of very short pulse duration
(1–10 ns) or an ac discharge between two electrodes
where at least one of the electrodes is covered with a di-
electric [87.68]. The dielectric acts like a high value
impedance which prevents discharge current runaway
and tends to distribute the average current fairly uni-
formly across the surface area. The charge transferred
by the streamer to the dielectric essentially compensates
for the external electric field and limits the discharge
duration. Close examination shows that the current
terminations on the cathode dielectric are many mi-
crodischarges; the termination at the dielectric anode
is usually diffuse. The cathode spot diameters are typi-
cally only 200 µm so that with a peak current of 0.1A,
the current density is hundreds of A/cm
2
. However, be-
cause of the usually very low duty cycle, the neutral gas
temperature is close to ambient. The electron temper-
atures during the discharge pulse are estimated in the
range of 1–10 eV, so the DBD is a very non-equilibrium
plasma. The charge transferred to the dielectric on the
previous pulse strongly affects the next pulse. The high
field discharge is efficient at producing excitation, dis-
sociation, and ionization. Miniature DBDs are used in
plasma display panels; these are discharges between
electrode arrays coated with dielectric and separated
by typically 100 µm in a Penning gas mixture at about
500 Torr [87.69].
Part G 87.4