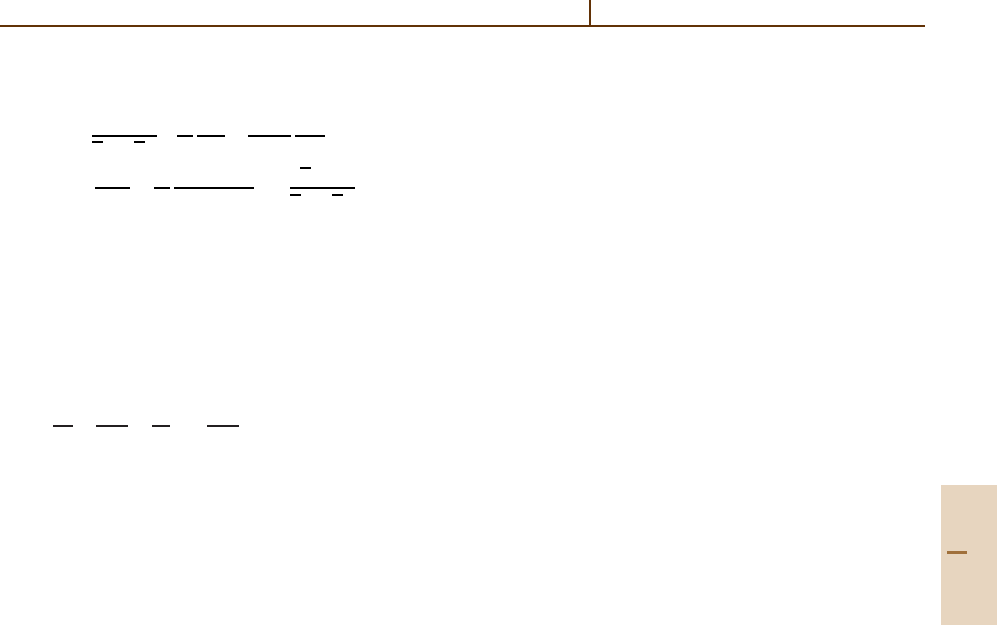
Aeronomy 84.5 Neutral, Ion and Electron Temperatures 1281
For a minor ion i diffusing through a major ion species j,
its velocity is given by
w
i
=−
k
B
T
i
/m
i
ν
i j
+ν
in
1
n
i
dn
i
ds
+
T
e
/T
i
n
e
dn
e
ds
(84.65)
+
m
i
g
k
B
T
i
+
1
T
i
d(T
e
+T
i
)
ds
−
ν
i j
w
j
ν
i j
+ν
in
.
For regions in which there are large gradients in the ion
or electron temperatures, thermal diffusion may also be
important in determining the ion density profiles, espe-
cially those of light ions such as H
+
and He
+
. Equations
for ion distributions in which thermal diffusion is in-
cluded have been presented by, for example, Schunk and
Nagy [84.71] and references therein.
84.5 Neutral, Ion and Electron Temperatures
The temperature distribution in planetary thermo-
spheres/ionospheres can be modeled by solving the
equation for conservation of energy, which, in simplified
form in the vertical direction is
n
m
N
2
k
B
∂T
m
∂t
−
∂
∂z
κ
m
∂T
m
∂z
=Q
m
−L
m
(84.66)
where N is the number of degrees of freedom (3 for
an atom, and 5 for a diatomic molecule), the subscript
m refers to the neutrals, ions or electrons, κ
m
is the
thermal conductivity, Q
m
is the volume heating rate, and
L
m
is the volume cooling rate. If horizontal variations
are considered, the model becomes multidimensional
and advective terms must be added to the equations.
The T
n
are also affected by compression or expansion
due to subsidence or upwelling, respectively. Viscous
heating may be a factor where there are local regions
of intense energy input, such as in auroral arcs. These
terms are not shown in the energy equation above, but
may be found in standard aeronomy texts, such as Banks
and Kockarts [84.72]orRees [84.44]andSchunk and
Nagy [84.73]. For planets with intrinsic magnetic fields,
the electrons and ions are constrained to move along
magnetic field lines, and the second term on the left-hand
side of (84.66) must be multiplied by a factor sin
2
I.
The neutral thermospheres of planets are mostly
heated by absorption of solar radiation in the 10 to
2000 Å range, although on planets with powerful au-
roras, electron precipitation may be an important source
of heat. Absorption of EUV radiation
100–1000 Å
largely results in ionization of the major thermospheric
species, in which most of the excess energy is carried
away by the photoelectron. The photoelectron, however,
may produce further dissociation or excitation of neu-
tral species along the path to thermalization, and these
processes may result in neutral heating. Photons near
and longward of ionization thresholds in the FUV may
lose their energy in photodissociation, in which the ex-
cess energy of the photon appears as kinetic or internal
energy of the fragments.
Chemical reactions that follow ionization or dissoci-
ation release much of the absorbed solar energy as heat.
Although the partitioning of kinetic energy released be-
tween the product species can be determined easily by
conservation of energy and momentum, the fraction of
energy that appears as internal or kinetic energy must
be determined by measurements or theoretical calcula-
tions. If vibrationally or electronically excited states are
produced in these interactions, however, the energy may
be radiated to space, thus producing cooling. This may
occur promptly if the radiative lifetime is short, or sub-
sequent to an energy transfer process from a long-lived
metastable species to a species for which radiation to
a lower state is allowed. If the metastable species is
quenched, however, its energy can also appear as heat.
Thus the energy partitioning in chemical reactions and
in the interactions of photons and photoelectrons with
atmospheric species is important in understanding the
temperature structure of thermospheres.
A heating efficiency is often defined as the frac-
tion of energy absorbed at a given altitude that appears
locally as heat. The heating efficiencies are in the range
30–40% in the terrestrial lower thermosphere. Above
200 km, the heating efficiency decreases because the
energy of the important metastable species O(
1
D) is
lost as radiation rather than by quenching [84.74]. The
heating efficiencies in the thermospheres of Venus and
Mars are about 20% from 100 to 200 km [84.75, 76],
and on Titan, they range from 20 to 30% from 800
to 2000 km. A column averaged heating efficiency
for the Jovian thermosphere has been computed as
53% [84.77].
On Venus and Mars, CO
2
is the major absorber of far
UV radiation, whereas on the Earth, O
2
plays that role.
In the F
1
regions of the ionospheres of the terrestrial
planets, dissociative recombination of molecular ions
tends to be the major source of heating. Below the F
1
peak, photodissociation and neutral-neutral reactions,
including quenching of metastable species, dominate.
Since CH
4
is a very strong absorber, the major heating
Part G 84.5