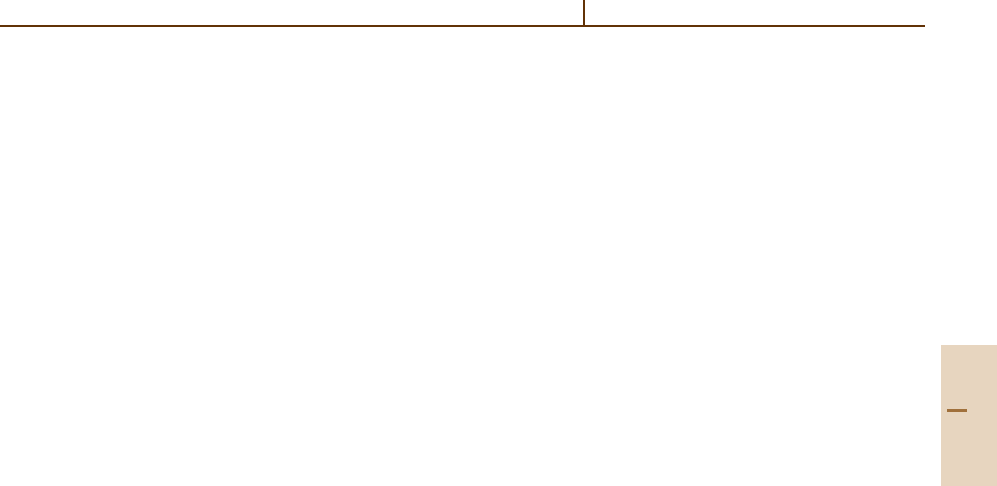
Ion–Molecule Reactions 67.4 New Directions: Complexity and Imaging 991
67.3.6 Energy Dependent Total Cross
Sections
Although non-state-selected cross sections, denoted
σ(v), lack information about product energy disposal,
important features of the potential energy surface can
be obtained from their measurement. Guided ion beam
methods have been especially important in the deter-
mination of accurate cross sections, particularly those
that employ a supersonic beam rather than a gas as a
neutral target. Energy-dependent cross sections provide
crucial information on ion and neutral thermochemistry
through accurate measurements of reaction thresholds,
and help to elucidate important potential surface fea-
tures such as thresholds, barriers, and crossings [67.30].
A particularly illustrative example of threshold forma-
tion concerns the reaction N
+
(
3
P) + H
2
→ NH
+
+ H
and its isotopic variants. This reaction is important at
low (< 0.1 eV) collision energies as the first step in
the chain reaction that leads to formation of ammo-
nia in dense interstellar clouds, but the bond energy of
NH
+
is uncertain enough to prevent knowing whether
the above reaction is endothermic or exothermic. Re-
cent total cross section measurements on the isotopic
variant N
+
(
3
P) + D
2
→ ND
+
+ D at very low col-
lision energies now appear to answer this question
[67.28]. Figure 67.8 shows experimental results per-
formed on two different instruments, one a guided
ion beam-crossed neutral beam machine and the other
a guided merged-beam apparatus. The data show a
very clear threshold at 15 meV, demonstrating that
the reaction is endothermic and allowing for a more
accurate estimate of the ND
+
bond energy. This
experiment shows that the high energy resolution af-
forded in total cross section measurements employing
crossed and merged beams, rather than thermal col-
lision cells, can answer thermochemical questions of
importance in a wide variety of applications, includ-
ing astrophysics, combustion, electrical discharges, and
atmospheric processes.
67.4 New Directions: Complexity and Imaging
The crossed beam technique provides the precise kine-
matic definition required to extract the most intimate
details of reactive collisions. Although the highest res-
olution examples of the technique involve systems
with three or four atoms, several recent examples of
systems of greater complexity have appeared in the
literature. The multiply-charged CF
2+
2
+ D
2
system
[67.31] is appreciably more complex experimentally
than its singly-charged analog, owing to the possibility
of forming two charged fragments with correlated prod-
uct distributions. The nine-atom C
2
H
+
2
+ CH
4
system
has been studied previously with guided ion beam-
gas cell methods under conditions where the reactant
ions are produced by multiphoton ionization [67.32].
Those early experiments, which yielded total reaction
cross sections for vibrational state-selected ions, gave
significant indications of mode-selectivity in complex
reactions. A more recent study [67.33], conducted with-
out reactant state-selection or product state analysis,
maps out product angular distributions and disposal of
energy in relative translation with ion trapping tech-
niques that measure longitudinal velocity components
directly and provide upper bounds on the transverse
velocity components.
The majority of extant crossed beam experimen-
tal studies construct three-dimensional velocity space
distributions with a series of one-dimensional sections
through the full distribution such that, in any interval of
time, only a single detection element in velocity space
can be measured. Recent advances in imaging methods
[67.34–36], in which many velocity space elements can
be observed in a single time interval, promise to increase
the sensitivity of the crossed beams method by orders
of magnitude. The signal gains that can be achieved
with the implementation of such multiplex advantage
methods will allow the lower reactant and product sig-
nal levels associated with increasing state-specification
and/or system complexity to be tolerated without undue
increases in data acquisition times.
Figure 67.9 shows a schematic of an instrument that
illustrates the imaging principle.
As indicated in the diagram, the locus of points of
reaction products with a constant center of mass speed
is a sphere whose radius increases with time. Individ-
ual quantum states of a given reaction product form a
set of nested spheres in velocity space. Projecting this
set of product spheres onto a detection plane allows all
product velocity elements to be observed in a single
time window. Application of the inverse Abel trans-
form [67.37] allows the full three-dimensional velocity
distribution to be extracted from the two-dimensional
projection on the detection plane. Velocity focusing
methods [67.38] allow reaction products originating
from spatially distinct regions of the collision volume
Part E 67.4