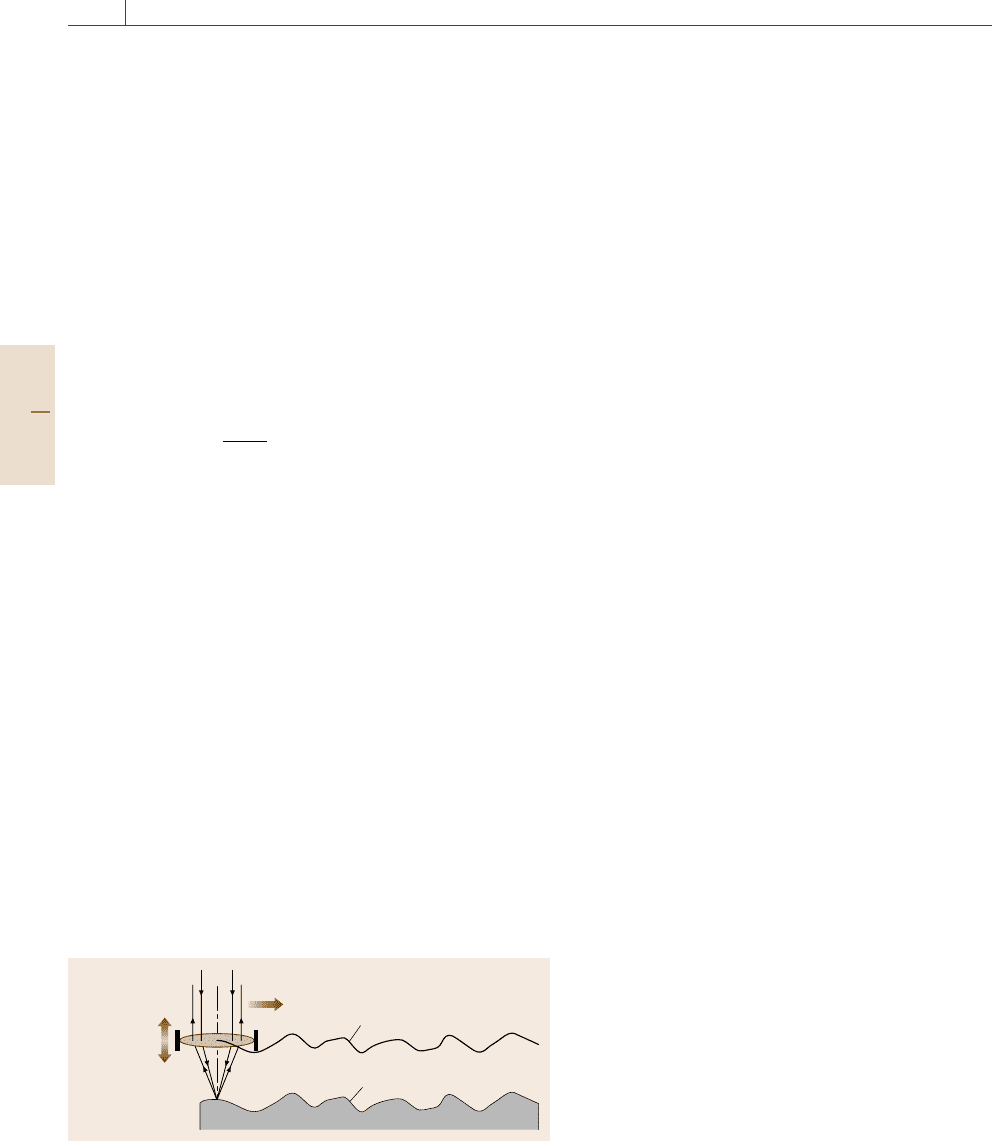
316 Part B Chemical and Microstructural Analysis
6.2.2 Optical Techniques
Many different measuring instruments based on optical
techniques exist [6.191, 193, 237, 238]. In this section,
the three most important ones are described: optical
stylus profilometry, confocal microscopy, and interfer-
ometry. Some issues are of importance to all optical
microscopy methods [6.239].
•
Material response: optical probing is only possi-
ble when a signal above the detector threshold is
received, which is determined by the material’s re-
flectivity.
•
Lateral resolution: this is limited by light diffrac-
tion. For an instrument numerical aperture NA and
a light wavelength λ, the limit d is given by [6.196]
d =
1.22λ
NA
. (6.44)
•
Maximum detectable slope: this depends on the kind
of reflection (specular or diffused), which in turn de-
pends on the surface topography and material, as
well as on the objective working distance and nu-
merical aperture.
•
Wavelength of full-amplitude modulation: this
quantity is intended as the maximum aspect ratio of
a measurable surface structure.
Optical Stylus Profilometry
Optical styli for profilometry can be based on the auto-
focusing signal of a laser beam detector. A laser beam
with a spot diameter of about 1 μm is focused onto
a point on the surface through a lens characterized by
a high numerical aperture (NA). The scattered light is
collected by the same lens on a focus detector, which
operates a control system. When the detector moves
horizontally, the controller, normally piezoelectric,
modifies the distance of the lens from the surface so as
to keep the beam focused. Consequently, the movement
Piezodrive
system
Lens trajectory
Profile
Fig. 6.21 Operating principle of the autofocusing method (after
[6.237])
of the lens follows the surface at a constant separation
distance, and its trajectory describes the surface profile,
as shown in Fig. 6.21. This kind of instrument is similar
in use to conventional stylus instruments, with vertical
resolution of approximately 5 nm. The optical method
is noncontacting, which allows measurements on soft
surfaces. However, this kind of instrument is connected
to some problems related to achieving a useful reflec-
tion signal from surfaces that are shiny or transparent
to the laser beam. The measurements obtained with the
autofocusing method do not always correlate very well
with those obtained with the stylus method [6.235,237],
as the optical method tends to overestimate the peak
heights and the stylus method to underestimate the val-
ley heights of the surface. The optical stylus method
was found to work well on very flat samples, but when
measuring roughnesses below 1 μs it was very prone to
error. The maximum detectable slope using an autofo-
cusing stylus instrument is approximately 15
◦
.
Confocal Microscopy
Confocal microscopy is an optical technique based on
the focus detection principle. It is routinely applied in
biological sciences, where relatively thick biological
samples, such as cells in tissue, are investigated using
fluorescence. However, it is also suitable for 3-D topog-
raphy assessment, when the reflected light is detected
rather than the emitted fluorescence. Here, the technique
is presented with reference to the reflection mode of
operation with a laser light source. The working prin-
ciple is easily seen by referring to Fig. 6.22, where key
components and optical ray diagrams are sketched (the
scanner which moves the laser spot on the surface is not
shown) [6.239].
Confocality consists in that both the light source
pinhole P1 and the detector pinhole P2 are focused on
the specimen. In laser scanning confocal microscopy
one surface picture element (pixel) is imaged at a time.
The final image is therefore built up sequentially. This
has relevant consequences for the application of this
technique to topography measurement; the measure-
ment time is negatively affected while the maximum
detectable surface slope is increased. Topography is re-
constructed as a stack of vertical optical sections, in
a fashion similar to computer tomography. In other
words, it is built by overlapping a number of opti-
cal slices with normal vectors aligned with the optical
axis. A single optical slice contribution to the final
topography is given by all of the pixels where re-
flection occurs. The two pinholes shown in Fig. 6.22
allow, in principle, the detection of light back from
Part B 6.2