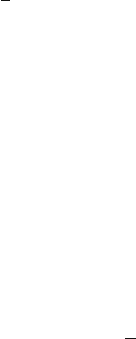
10.10 The LHC and the Search for the Higgs Boson 305
quark-gluon plasma can help to understand the origin of particles such as the proton
and the neutron, and may also have important implications for our understanding of
cosmology.
10.10 The LHC and the Search for the Higgs Boson
As shown in Chap. 9, the Standard Model of electroweak interactions has suc-
cessfully gathered an impressive amount of experimental confirmations. Indeed,
high-precision measurements and a thorough verification of the Standard Model
were performed in 1989 by the four LEP experiments at CERN (ALEPH, DELPHI,
L3 and OPAL) and the SLD experiment at Stanford. Nevertheless, the neutral Higgs
boson which is an indispensable ingredient in the Standard Model, used to explain
the origin of the masses, is still eluding experimental discovery. As described
in Sect. 11.5, the Higgs boson is introduced through the spontaneous symmetry
breaking mechanism responsible for generating masses of all known particles.
The advent of the Large Hadron Collider (LHC) at CERN (see Sect. 3.3), the
highest-energy particle accelerator in the world, allows one to extend the search
for the existence of the Higgs boson far beyond what was achieved by previous
experiments. In addition, new particles predicted by extensions of the Standard
Model, for example, the supersymmetric models (Sect. 13.2), are searched for at
LHC.
On September 10, 2008, the proton beams were successfully circulated in the
main LHC rings, but 9 days later, operations were halted due to a serious fault. After
a long repair and test period, the LHC was restarted in March 2010, and each beam
was accelerated up to 3.5 TeV (i.e., half of the design nominal beam energy). The
first beam collisions at a center-of-mass energy (
p
s) of 7 TeV were immediately
recorded by the detectors installed around the four LHC collision points; these
detectors are ATLAS, CMS, ALICE and LHCb. The first two experiments are large
omnipurpose detectors, while ALICE is mainly devoted to the search for the quark-
gluon plasma state (Sect. 10.9.1); the LHCb experiment is instead designed for high
statistic studies of CP violation (Sect.12.5). LHC will likely continue to operate at
this energy for a few years, but with an ever increasing instantaneous luminosity (in
2011, reaching 3:3 10
33
cm
2
s
1
). LHC will not likely be operated at its design
collision energy of 14 TeV until 2014.
On the experimental side, a proof of the high quality reached by the LHC
detectors is, for instance, the invariant mass spectrum of opposite-sign muon pairs
obtained with the CMS data, see Fig. 10.25. A muon pair can be produced by the
combination of background events, or from a Drell–Yan process, see Sect. 7.14.2.
Referring to Fig. 7.20, at the LHC c.m. energy, the exchange of a Z
0
, in addition to
the , is also possible. In the case of the Drell–Yan mechanism, the q
q vector meson
resonances and the Z
0
on-mass shell are observed as peaks visible in Fig. 10.25.