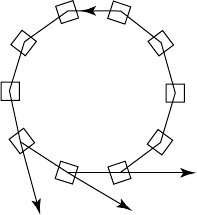
22.3 Synchrotron X-ray sources 337
SR is produced tangentially every time the particle beam changes
direction in bending magnets or insertion devices (Fig. 22.4). As a result
of relativity, it is strongly concentrated in a single forward direction, giv-
ing a very highly collimated beam of radiation. It is almost completely
polarized in the plane of the storage ring, has a very high intensity
compared with conventional X-ray sources, and covers a continuous
wide spectrum from infrared to hard X-rays (undulators give a jagged
stepped X-ray spectrum), with a maximum photon energy (minimum
wavelength) dictated by the operating conditions. For the purposes of
X-ray crystallography, we can think of a synchrotron storage ring in sim-
ple terms as a large device that exploits relativity to convert microwave
energy into X-rays by a massive doppler shift. Any wavelength can
be selected from the broad spectrum by a monochromator, or the con-
tinuous ‘white’ X-ray spectrum can be used for the Laue diffraction
technique, which is not discussed in this book.
Electron beam
Magnets
S
nchrotron radiation
Fig. 22.4 The principle of operation of a
synchrotron storage ring.
The very high intensity, several orders of magnitude greater than
from conventional sources, is the most obvious and desirable feature
of SR X-rays. It allows diffraction patterns to be measured quickly, even
from tiny crystals (down to micrometre dimensions, depending on the
chemical composition and crystal quality of the sample), or from other
samples giving relatively weak diffraction as a result of structural faults
such as disorder. Obviously this is useful when larger single crystals
can not be obtained, and individual powder grains can be treated as
single crystals, though therequirements of crystalmounting and diffrac-
tometer mechanical precision are demanding; it is also an advantage
for chemically unstable and sensitive materials, and makes it possible
(in combination with the pulsed nature of SR and the use of lasers) to
investigate short-lived excited states.
The advantages of the high intensity of SR are further enhanced
by the high degree of collimation, which makes individual reflections
from single-crystal samples stand out moreclearly from the background
because of their sharper profiles; these are dictated largely by the sam-
ple quality rather than a non-parallel incident X-ray beam. This can
also help in the spatial resolution of reflections from a sample with a
large unit cell, or if a short wavelength is selected. A short wavelength
gives access to higher-resolution data for charge-density studies, can
reduce some systematic errors such as absorption and extinction, and
can allow more of the diffraction pattern to be measured from a sample
in a diamond anvil high-pressurecell or other special environment. Con-
versely, a longer wavelength spreads out a dense diffraction pattern for
a large structure. A particular wavelength might also be chosen to min-
imize or to maximize special effects such as anomalous scattering. Bent
monochromators and mirrors (giving total external reflection of X-rays
at a glancing angle) can be used to focus and concentrate the available
X-rays to match the sample size, so that the high flux is more effectively
usedashigh brilliance (flux in a given cross-sectionalareaor solid angle).
The pulsed nature of SR is exploited in special time-resolved stud-
ies, but is not relevant to most crystallographic users. The polarization