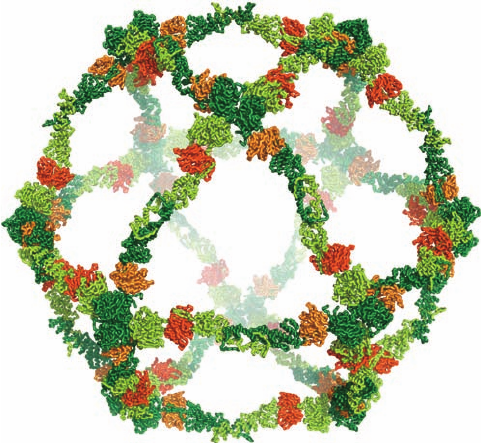
ture of the Sec13/31 cage (Fig. 12-70) to yield the model for
the COPII cage drawn in Fig. 12-72.
It is instructive to consider the similarities and differ-
ences between COPII and clathrin cages. Both consist of
seven-bladed  propellers and ␣ solenoids. In COPII cages,
all such motifs participate in forming its edges with four
Sec31  propellers associating to form each of its vertices.
In contrast, clathrin cages are constructed entirely from
their ␣ solenoidal segments with three such segments asso-
ciating to form each of its vertices and with their  propeller
motifs located in the interior of the cage, where they inter-
act with adapter proteins. Moreover, the ⬃40-Å-diameter
edges of COPII cages each consist of a double layer of
␣ solenoids, whereas the ⬃120-Å-diameter edges of clathrin
cages each consist of the interdigitated ␣ solenoidal seg-
ments from four triskelions. Evidently, evolution has
molded the similar components of these cages to different
functions. Sequence analysis of COPI coat proteins have
identified ␣ solenoid and  propeller motifs, which suggests
that the clathrin-, COPI-, and COPII-coated vesicles arose
from the same proto-coatamer.
The C-terminal segment of Sec31, which is not present
in the forgoing X-ray structures, contains an apparently
unstructured Pro-rich segment (residues 770–1110 are
20% Pro) that has been implicated in binding the
Sar1–Sec23/24 complex (which initiates vesicle budding
by binding to the cytoplasmic regions of cargo TM pro-
teins). Based on the X-ray structure of the Sar1–Sec23/24
complex and the fact that a cuboctahedral Sec13/31 cage
has has 48 binding sites for this complex, it would appear
that the Sar1–Sec23/24 complex forms a 50-Å-thick layer
beneath the surface of the COPII cage. Indeed, cryo-EM
studies on COPII vesicles assembled from purified
Sec13/31 and Sec23/24 complexes reveal that the Sec23/24
complexes form a cage that is concentric to and inside the
Sec13/31 cage.
g. Proteins Are Directed to the Lysosome by
Carbohydrate Recognition Markers
How are proteins in the ER selected for transport to the
Golgi apparatus and from there to their respective mem-
branous destinations? A clue as to the nature of this
process is provided by the human hereditary defect known
as I-cell disease (alternatively, mucolipidosis II), which in
homozygotes is characterized by severe progressive psy-
chomotor retardation, skeletal deformities, and death by
age 10.The lysosomes in the connective tissue of I-cell dis-
ease victims contain large inclusions (after which the dis-
ease is named) of glycosaminoglycans and glycolipids as a
result of the absence of several lysosomal hydrolases.These
enzymes are synthesized on the RER with their correct
amino acid sequences but, rather than being dispatched to
the lysosomes, are secreted into the extracellular medium.
This misdirection results from the absence of a mannose-6-
phosphate recognition marker on the carbohydrate moi-
eties of these hydrolases because an enzyme required for
mannose phosphorylation fails to recognize the lysosomal
proteins. The mannose-6-phosphate residues are normally
bound by a receptor in the coated vesicles that transport
lysosomal hydrolases from the Golgi apparatus to the lyso-
somes (Section 23-3Bj). Other glycoproteins are directed
to their intracellular destinations by similar carbohydrate
markers.
h. ER-Resident Proteins Have the C-Terminal
Sequence KDEL
Most soluble ER-resident proteins in mammals have
the C-terminal sequences KDEL (HDEL in yeast),
KKXX, or KXKXXX (where X represents any amino acid
residue), whose alteration results in the secretion of the
resulting protein. By what means are these proteins selec-
tively retained in the ER? Since many ER-resident pro-
teins freely diffuse within the ER, it seems unlikely that
they are immobilized by membrane-bound receptors
within the ER. Rather, it has been shown that ER-resident
proteins, as do secretory and lysosomal proteins, readily
leave the ER via COPII-coated vesicles but that ER-resident
proteins are promptly retrieved from the Golgi and returned
to the ER in COPI-coated vesicles. Indeed, coatomer binds
the Lys residues in the C-terminal KKXX motif of trans-
membrane proteins, which presumably permits it to gather
these proteins into COPI-coated vesicles. Furthermore,
genetically appending KDEL to the lysosomal protease
cathepsin D causes it to accumulate in the ER, but it nev-
ertheless acquires an N-acetylglucosaminyl-1-phosphate
group, a modification that is made in an early Golgi com-
partment. Presumably, a membrane-bound receptor in a
post-ER compartment binds the KDEL signal and the
Section 12-4. Membrane Assembly and Protein Targeting 439
Figure 12-72 Molecular model of the COPII cage viewed
approximately along its 3-fold axis. Its 48 Sec13/31 subunits are
drawn in worm form colored as in Fig. 12-71. Four Sec31 
propellers associate to form each vertex of the cuboctahedral
cage with the remaining portions of the heterotetrameric
Sec13/31 assembly units forming its edges.The inner diameter of
the cage is ⬃520 Å. [Courtesy of Jonathan Goldberg, Memorial
Sloan-Kettering Cancer Center, New York, New York.]
JWCL281_c12_386-466.qxd 6/9/10 12:06 PM Page 439