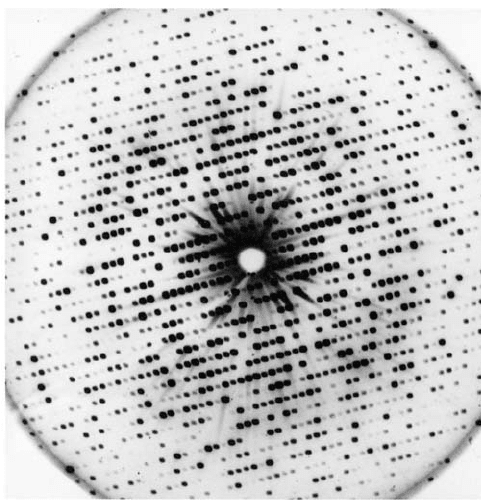
3 GLOBULAR PROTEINS
Globular proteins comprise a highly diverse group of sub-
stances that, in their native states, exist as compact spher-
oidal molecules. Enzymes are globular proteins, as are
transport and receptor proteins. In this section we consider
the tertiary structures of globular proteins. However, since
most of our detailed structural knowledge of proteins, and
thus to a large extent their function, has resulted from X-
ray crystal structure determinations of globular proteins
and, more recently, from their nuclear magnetic resonance
(NMR) structure determinations, we begin this section
with a discussion of the capabilities and limitations of these
powerful techniques.
A. Interpretation of Protein X-Ray
and NMR Structures
X-ray crystallography is a technique that directly images
molecules. X-rays must be used to do so because, accord-
ing to optical principles, the uncertainty in locating an ob-
ject is approximately equal to the wavelength of the radia-
tion used to observe it (covalent bond distances and the
wavelengths of the X-rays used in structural studies are
both ⬃1.5 Å; individual molecules cannot be seen in a
light microscope because visible light has a minimum
wavelength of 4000 Å).There is, however, no such thing as
an X-ray microscope because there are no X-ray lenses.
Rather, a crystal of the molecule to be imaged is exposed
to a collimated beam of X-rays and the consequent dif-
fraction pattern is recorded by a radiation detector or,
now infrequently, on photographic film (Fig. 8-35). The
X-rays used in structural studies are produced by laboratory
X-ray generators or, increasingly often, by synchrotrons, a
type of particle accelerator that produces X-rays of far
greater intensity. The intensities of the diffraction maxima
(darkness of the spots on a film) are then used to construct
mathematically the three-dimensional image of the crystal
structure through methods that are beyond the scope of
this text. In what follows, we discuss some of the special
problems associated with interpreting the X-ray crystal
structures of proteins.
X-rays interact almost exclusively with the electrons in
matter,not the far more massive nuclei.An X-ray structure
is therefore an image of the electron density of the object
under study. Such electron density maps may be presented
as a series of parallel sections through the object. On each
section, the electron density is represented by contours
(Fig. 8-36a) in the same way that altitude is represented by
the contours on a topographic map. A stack of such sec-
tions, drawn on transparencies, yields a three-dimensional
electron density map (Fig. 8-36b). Modern structural analy-
sis, however, is carried out with the aid of computers that
graphically display electron density maps that are con-
toured in three dimensions (Fig. 8-36c).
a.
Most Protein Crystal Structures Exhibit Less than
Atomic Resolution
The molecules in protein crystals, as in other crys-
talline substances, are arranged in regularly repeating
three-dimensional lattices. Protein crystals, however, dif-
fer from those of most small organic and inorganic mole-
cules in being highly hydrated; they are typically 40 to
60% water by volume. The aqueous solvent of crystalliza-
tion is necessary for the structural integrity of the protein
crystals, as J.D. Bernal and Dorothy Crowfoot Hodgkin
first noted in 1934 when they carried out the original
X-ray studies of protein crystals. This is because water is
required for the structural integrity of native proteins
themselves (Section 8-4).
The large solvent content of protein crystals gives them
a soft, jellylike consistency, so that their molecules often
lack the rigid order characteristic of crystals of small mole-
cules such as NaCl or glycine. The molecules in a protein
crystal are typically disordered by more than an angstrom,
so that the corresponding electron density map lacks infor-
mation concerning structural details of smaller size. The
crystal is therefore said to have a resolution limit of that
size. Protein crystals typically have resolution limits in the
range 1.5 to 3.0 Å, although some are better ordered (have
higher resolution, that is, a lesser resolution limit) and
many are less ordered (have lower resolution).
Since an electron density map of a protein must be in-
terpreted in terms of its atomic positions, the accuracy and
Section 8-3. Globular Proteins 241
Figure 8-35 X-ray diffraction photograph of a single crystal of
sperm whale myoglobin. The intensity of each diffraction
maximum (the darkness of each spot) is a function of the
myoglobin crystal’s electron density. The photograph contains
only a small fraction of the total diffraction information available
from a myoglobin crystal. [Courtesy of John Kendrew, Cambridge
University, U.K.]
JWCL281_c08_221-277.qxd 2/23/10 1:58 PM Page 241