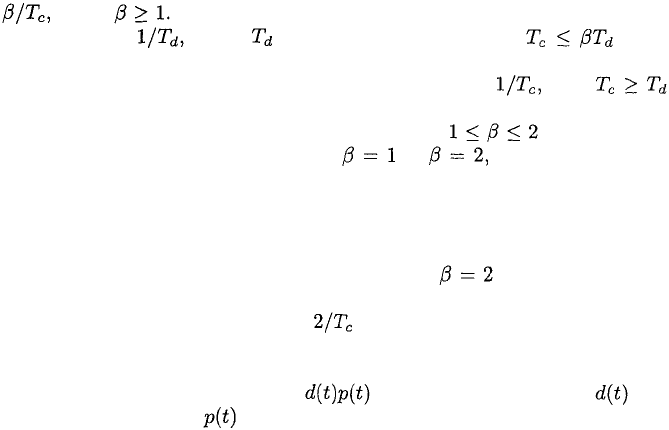
318
CHAPTER 6.
CODE-DIVISION MULTIPLE ACCESS
the maximal-ratio combining of the parallel correlator outputs, each of which is
associated with a different carrier. Bit error probabilities are determined subse-
quently for ideal multicarrier and single-carrier systems with lossless diversity
combining in the presence of white Gaussian noise and Rayleigh fading.
Multicarrier Direct-Sequence System
A typical multicarrier system divides a spectral band of bandwidth W into L
frequency channels or subchannels, each of bandwidth W/L. The carrier asso-
ciated with a subchannel is called a subcarrier. In one type of system, which is
diagrammed in Figure 6.9, this bandwidth is approximately equal to the coher-
ence bandwidth because a larger one would allow frequency-selective fading in
each subchannel, while a smaller one would allow correlated fading among the
subcarriers [8], [9]. It is assumed that the spacing between adjacent subcarriers
is where Equation (5-57) indicates that the coherence bandwidth
is approximately where is the delay spread. Thus, is re-
quired to ensure that each subcarrier signal is subject to independent fading.
If the bandwidth of a subcarrier signal is on the order of then
is required for the subcarrier signals to experience no significant frequency se-
lectivity. The two preceding inequalities imply that is required. If
the chip waveforms are rectangular and or then the subcarrier
frequencies are orthogonal, which can be verified by a calculation similar to that
leading to (3-59). Although the orthogonality prevents self-interference among
the subcarrier signals, its effectiveness is reduced by multipath components and
Doppler shifts. One may use bandlimited subcarrier signals to minimize the
self-interference without requiring orthogonality. If and the chip wave-
forms are rectangular, then the spectral mainlobes of the subcarrier signals have
no overlap. Furthermore, a spacing of limits the significant multiple-access
interference in a subchannel to subcarrier signals from other users that have the
same subcarrier frequency.
In the transmitter, the product of the data modulation and
the spreading waveform simultaneously modulates L subcarriers, each of
which has its frequency in the center of one of the L spectral regions, as illus-
trated in Figure 6.9(a). The receiver has L parallel demodulators, one for each
subcarrier, the outputs of which are suitably combined, as indicated in Figure
6.9(b). The total signal power is divided equally among the L subcarriers. The
chip rate and, hence, the processing gain for each subcarrier of a multicarrier
direct-sequence system is reduced by the factor
L. However, if strong interfer-
ence exists in a subchannel, the gain used in maximal-ratio combining is small.
Alternatively, the associated subcarrier can be omitted and the saved power
redistributed among the remaining subcarriers. Error-control codes and inter-
leaving can be used to provide both time diversity and coding gain. Since the
spectral regions are defined so that the fading in each of them is independent and
frequency nonselective, rake combining is not possible, but the frequency diver-
sity provided by the regions can be exploited in a diversity combiner. Whether
or not the diversity gain exceeds that of a single-carrier system using the entire