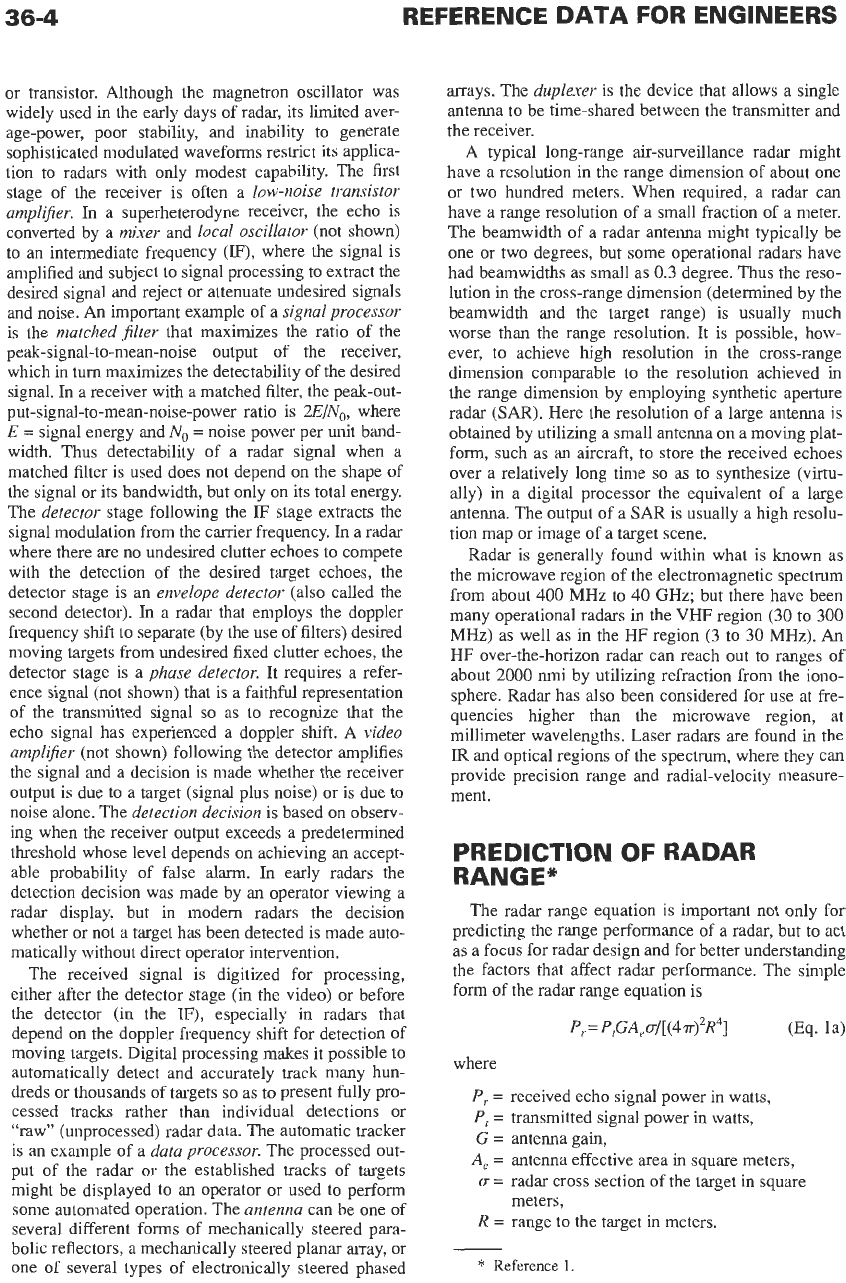
or transistor. Although the magnetron oscillator was
widely used in the early days of radar, its limited aver-
age-power, poor stability, and inability to generate
sophisticated modulated waveforms restrict its applica-
tion to radars with only modest capability. The first
stage of the receiver is often a
low-noise transistor
amplger.
In
a superheterodyne receiver, the echo is
converted by a
mixer
and
local oscillator
(not shown)
to an intermediate frequency
(IF),
where the signal is
amplified and subject to signal processing to extract the
desired signal and reject or attenuate undesired signals
and noise.
An
important example of a
signal processor
is
the
matched filter
that maximizes the ratio
of
the
peak-signal-to-mean-noise output
of
the receiver,
which in turn maximizes the detectability of the desired
signal.
In
a receiver with a matched filter, the peak-out-
put-signal-to-mean-noise-power ratio is
=/No,
where
E
=
signal energy and
No
=
noise power per unit band-
width. Thus detectability of a radar signal when a
matched filter is used does not depend on the shape
of
the signal or its bandwidth, but only on
its
total energy.
The
detector
stage following the
IF
stage extracts the
signal modulation from the carrier frequency.
In
a radar
where there are
no
undesired clutter echoes to compete
with the detection of the desired target echoes, the
detector stage is an
envelope detector
(also called the
second detector).
In
a radar that employs the doppler
frequency
shift
to separate (by the use of filters) desired
moving targets from undesired fixed clutter echoes, the
detector stage is a
phase detector.
It requires a refer-
ence signal (not shown) that is a faithful representation
of the transmitted signal
so
as to recognize that the
echo signal has experienced a doppler shift. A
video
ampl@er
(not shown) following the detector amplifies
the signal and a decision is made whether the receiver
output is due to a target (signal plus noise) or is due to
noise alone. The
detection decision
is based on observ-
ing when the receiver output exceeds a predetermined
threshold whose level depends on achieving an accept-
able probability
of
false alarm.
In
early radars the
detection decision was made by an operator viewing a
radar display, but
in
modem radars the decision
whether
or
not a target has been detected is made auto-
matically without direct operator intervention.
The received signal
is
digitized for processing,
either after the detector stage
(in
the video) or before
the detector (in the
IF),
especially in radars that
depend
on
the doppler frequency shift for detection
of
moving targets. Digital processing makes it possible to
automatically detect and accurately track many hun-
dreds or thousands of targets
so
as to present fully pro-
cessed tracks rather than individual detections or
“raw” (unprocessed) radar data. The automatic tracker
is an example of a
data processor.
The processed out-
put of the radar or the established tracks of targets
might be displayed to an operator or used to perform
some automated operation. The
antenna
can be one of
several different forms of mechanically steered para-
bolic reflectors, a mechanically steered planar array, or
one
of
several types
of
electronically steered phased
arrays. The
duplexer
is the device that allows a single
antenna to be time-shared between the transmitter and
the receiver.
A typical long-range air-surveillance radar might
have a resolution in the range dimension of about one
or
two hundred meters. When required, a radar can
have a range resolution of a small fraction of a meter.
The beamwidth of a radar antenna might typically be
one or two degrees, but some operational radars have
had beamwidths as small as
0.3
degree. Thus the reso-
lution in the cross-range dimension (determined by the
beamwidth and the target range) is usually much
worse than the range resolution.
It
is possible, how-
ever, to achieve high resolution
in
the cross-range
dimension comparable to the resolution achieved in
the range dimension by employing synthetic aperture
radar
(SAR).
Here the resolution of a large antenna is
obtained by utilizing a small antenna on a moving plat-
form, such as an aircraft, to store the received echoes
over a relatively long time
so
as to synthesize (virtu-
ally) in a digital processor the equivalent of a large
antenna. The output of a
SAR
is usually a high resolu-
tion map or image of a target scene.
Radar is generally found within what is known as
the microwave region of the electromagnetic spectrum
from about
400
MHz to
40
GHz; but there have been
many operational radars in the
VHF
region
(30
to
300
MHz) as well as
in
the HF region
(3
to
30
MHz).
An
HF over-the-horizon radar can reach out to ranges of
about
2000
nmi by utilizing refraction from the iono-
sphere. Radar has also been considered for use at fre-
quencies higher than the microwave region, at
millimeter wavelengths. Laser radars are found in the
IR
and optical regions of the spectrum, where they can
provide precision range and radial-velocity measure-
ment.
PREDICTION
OF
RADAR
RANGE*
The radar range equation is important not only for
predicting the range performance of a radar, but to act
as a focus for radar design and for better understanding
the factors that affect radar performance. The simple
form
of
the radar range equation is
where
P,.
=
received echo signal power in watts,
P,
=
transmitted signal power in watts,
G
=
antenna gain,
A,
=
antenna effective area in square meters,
m
=
radar cross section of the target in square
R
=
range
to
the target in meters.
meters,
*
Reference
1.