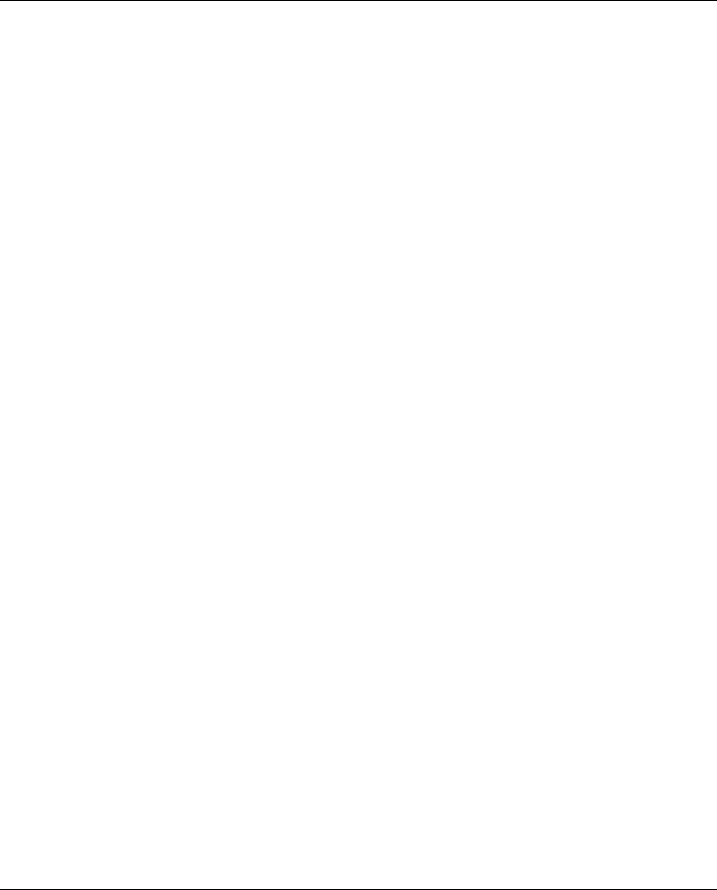
lysis, we performed flotation experiments according to protocol I with increasing
detergent-to-lipid ratios during lysis (Fig. 3). The silver stains in Fig. 3 show no
major differences in the distribution of the cytoskeletal and DRM marker proteins
within the density gradients of the three experiments, irrespective of whether
erythrocytes or ghosts are used as starting material. Therefore, this result does
not support the explanation of incomplete lysis for the cytoskeleton–DRM asso-
ciation.
To study the cytoskeleton–DRM interaction in more detail, we developed
another new method to efficiently and quickly separate cytoskeleton–DRM com-
plexes by non-equilibrium gradient centrifugation (NEGC). TX-100 lysates of
ghosts or erythrocytes are placed on top of a sucrose step gradient and subjected to
ultracentrifugation for 1 h at 38,000 g. Using this method, the TX-100-soluble
and cytosolic proteins can be recovered in the first two fractions of the gradient
comprising the loading zone and part of the 20% sucrose layer, whereas the cyto-
skeleton is present at the interphase between the 35% and the 60% sucrose layer.
Compared to the flotation method that needs at least 16 h of centrifugation, this
NEGC is a quick procedure with the respective advantages. Fig. 4A shows a typical
NEGC experiment of a ghost lysate. Most of the band 3 membrane protein and
band 6 are found in the first two fractions, and the major amounts of spectrin,
protein 4.1 and actin are present in fractions 9 and 10. A high percentage of the
DRM markers stomatin, flotillins, AChE, SM and about 50% of the membrane
cholesterol co-distribute together with the cytoskeleton in fractions 9 and 10 sup-
porting our view of an association of DRMs with the cytoskeleton. An aliquot of
fraction 9 was diluted, incubated with an alkaline buffer (pH 11) on ice to disrupt
the cytoskeleton and then subjected to a short ultracentrifugation step. The re-
sulting pellet and supernatant were analysed for the distribution of the cytoskeletal
and DRM markers. As expected, the cytoskeletal components were found in the
supernatant, whereas all the lipid and protein DRM markers were recovered in the
pellet (data not shown) indicating the presence of intact DRMs in this fraction. As
already shown in Fig. 1B, alkaline treatment disrupts the cytoskeletal interactions,
whereas DRM integrity is unaffected.
To dissolve DRMs, we performed an identical experiment as described in
Fig. 1A except that the lysis temperature was 371C. As expected, the DRM markers
are exclusively found in the TX-100-soluble fraction at the top of the gradient,
whereas the cytoskeleton is present in the bottom fraction demonstrating that the
Figure 3 DRM preparations are independent of the investigated lipid-to-detergent ratios. 50 ml
erythrocytes (A, B, and C) or ghosts (D, E, and F) are diluted with 100 ml (A, D), 250 ml(B,E)or
550 ml(C,F)andlysedwith150m l(A,D),300ml(B,E),or600ml (C, F) of ice-cold 2% Triton X-
100,10 mM EDTA, and proteinase inhibitors inTBS, incubated for 10 min on ice, and centrifuged
at 15,000xg for 20 min, 41C. The pellets were resuspended in cold 1% Triton X-100 containing
5 mM EDTA and proteinase inhibitors in TBS to give 300 ml. The sucrose gradients,
ultracentrif ugation, and gradient fractionation were performed as described in Fig. 1.The
following relative volumes of the fractions were analysed by 11% SDS-PAGE and silver
staining: 1.00 (fractions 1 and 2), 0.40 (fractions 3 ^5) and 0.25 (fractions 6^8). LD, I D and HD
indicate low-, intermediate-, and high-dens ity regions, respectively. Open triangles indicate the
positions of lipid raft marker proteins, ¢l led triangles indicate the positions of cytoskeletal
proteins.
U. Salzer et al.62