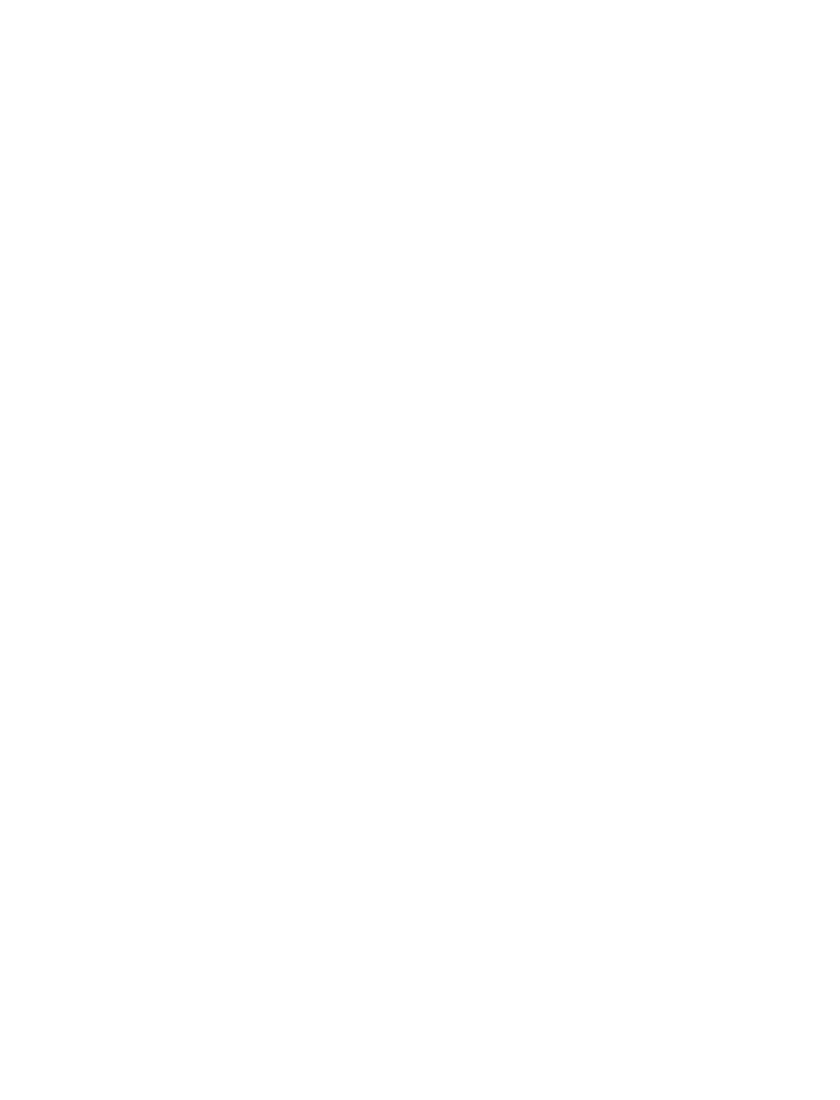
LITHIUM-ION BATTERIES 35.13
The LiNi
0.7
Co
0.2
Ti
0.005
Mg
0.005
O
2
material, engineered to offer improved safety through
reduced exotherm on decomposition, offers slightly lower capacity, 183 mAh/g on the first
deintercalation, and 165 mAh/ g reversibly, but with voltage similar to the other LiNi
1
⫺
x
Co
x
O
2
materials described.
Synthesis of Lithiated Metal Oxides. The synthesis of lithiated metal oxides, including
LiCoO
2
and LiMn
2
O
4
, has be achieved through a wide variety of routes, although those
practiced commercially use inexpensive starting materials, such as lithium carbonate, lithium
hydroxide and the metal oxide. The physical and electrochemical properties of the materials
may be controlled by the choice of starting materials and the preparation conditions.
18
The easiest to prepare is LiCoO
2
. Its thermodynamic stability results in the desired phase
after treatment of a range of lithium and cobalt reagents, including carbonates,
19
oxides,
20
hydroxides, nitrates,
21
and organic acid complexes,
22
in the appropriate ratio at high tem-
perature, 600
⬚C to 1100⬚C, in air. LiCoO
2
can be prepared in bulk at lower temperatures,
400
⬚C, from either cobalt nitrate
23
or the acetates.
24
Preparation of LiCoO
2
at 300⬚C has
been achieved from hydroxide mixtures.
25
LiCoO
2
has also been prepared via nonaqueous
routes from nitrates,
26
under hydrothemal conditions,
27
and as thin films by laser ablation
28
or spray pyrolysis.
29
An overview of the preparation and properties of lithium cobalt oxides
is available.
30
Lithium nickel cobalt oxides can be prepared using routes similar to those used in the
preparation of LiCoO
2
, although the properties of the material are more sensitive to the
preparative method. Preparations of lithium nickel cobalt oxides are designed to achieve
molecular mixing of the cobalt and nickel materials prior to their reaction. Lithium cobalt
nickel oxides have been prepared from lithium, nickel and cobalt hydroxide co-precipitates
from nitrate solutions, treated between 400
⬚C and 800⬚C after removal of excess water.
31
Another preparation includes treatment of Li
2
CO
3
, CoCO
3
and Ni(NO
3
)
2
6H
2
O at 400⬚C.
32
The electrochemical properties of LiMn
2
O
4
materials are sensitive to the preparative
method, motivating the development of preparations that yield single phase material with
controlled Li, Mn, and O stoichiometry with the desired structure. The effect of preparation
conditions on the properties of spinels prepared from Li
2
CO
3
and MnO
2
, such as electrolytic
MnO
2
(EMD), at 600⬚Cto900⬚C,
33
has been the subject of numerous investigations.
34
Ma-
terials have also been prepared from LiOH and
␥
-MnO
2
(CMD),
35
or Mn
2
O
3
,
36
towards
spinels with improved capacity retention.
35.2.3 Capacity Fade in C/ LiMn
2
O
4
Cells
Manganese-based materials continue to receive industrial and commercial interest because
of their low cost, benign environmental qualities,
37
good electrochemical properties, and
excellent safety properties, despite the higher capacity and improved high temperature sta-
bility possible when cobalt- or nickel-based materials are used. The complex chemistry of
the lithium manganese oxides has been the focus of many academic
38
and industrial studies.
The most significant differences in performance between typical manganese- and cobalt-
based cells are their lower capacity, their higher rate of capacity fade when cycled or stored
in the charged or discharged state, and the ability of spinel cells to sustain abuse, attributed
to the stability of
-MnO
2
to deoxygenation, relative to NiO
2
or CoO
2
.
Capacity fade in spinel cells is the result of multiple processes,
39
including those related
solely to the spinel material and others involving the interaction of spinel with the electrolyte
and negative electrode materials. Most fade mechanisms can be attributed to three factors:
40
1. Dissolution of Mn
2
⫹
into the electrolyte after disproportionation of Li
x
Mn
2
O
4
:
3
⫹
4
⫹
2
⫹
2Mn → Mn ⫹ Mn
(solid) (solid) (solution)