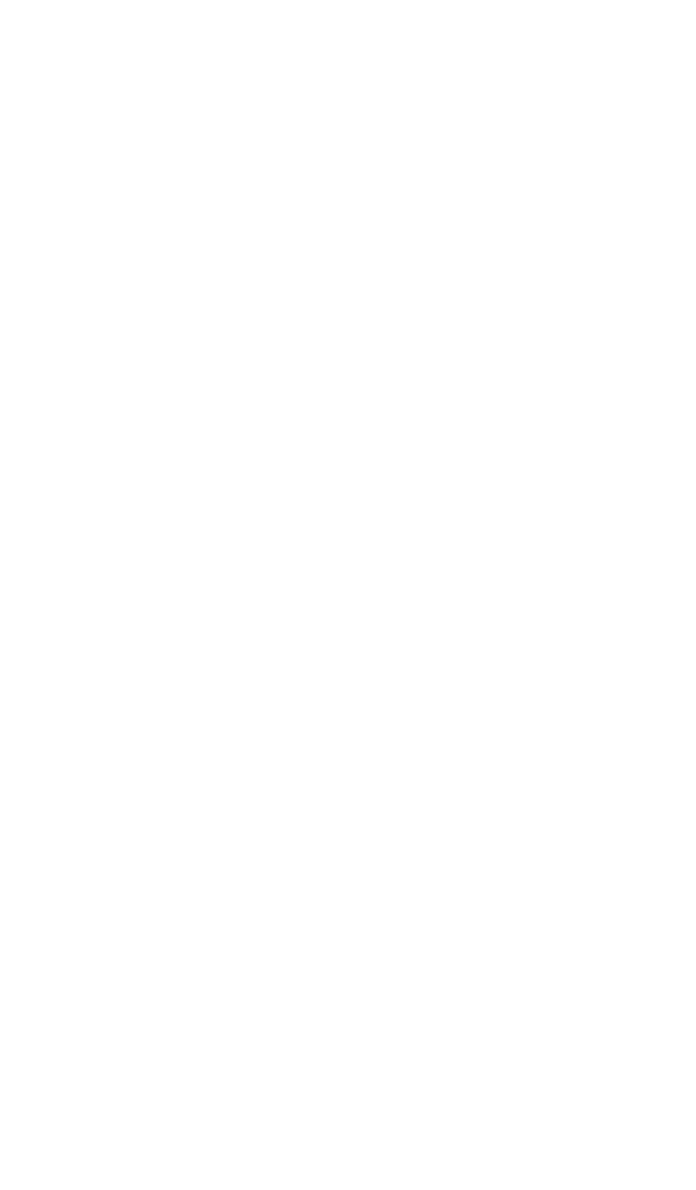
3 DIAMOND FILM DEPOSITION 1293
the keystones in CVD diamond deposition. Carbon produced from the gas phase
can take the form of many carbon materials. These carbon materials include
diamond, graphite, diamondlike carbon (DLC), carbynes, fullerenes, soot, naph-
thalene, and so on. However, the rate at which each of these materials is formed
depends on the total pressure of the system, substrate composition and temper-
ature, gas temperature, flowrates, and feedstock. Most low-pressure carbon-
producing systems produce nondiamond carbons in far greater abundance than
they produce diamond. It is necessary to shift deposition parameters in a direc-
tion that favors diamond carbon growth at the expense of the growth of the other
forms of carbon. However, this needs to be done while removing nondiamond
carbon from the system. The presence of nondiamond carbons tend to poison
diamond growth and interfere with the formation of good-quality diamond crys-
tals.
The etch rate of diamond in hydrogen and oxygen appears to be far less than
all other nondiamond carbons formed. This results in a diamond-rich carbon
deposit. If diamond is being formed at 10% of the rate of the other carbon
materials but is being etched at 1% of the rate of the other materials, then after
a period of time a net deposit of diamond will form. Diamondlike carbon films
or graphite will form if the substrate temperature is below 800
⬚C. The substrate
temperature must usually be somewhere between 800 and 1000
⬚C during CVD
diamond deposition. Diamond tends not to form if the substrate temperature
rises above 1100
⬚C. CVD diamond, single-crystal natural, and high-pressure
synthetic diamond graphitize and etch above this temperature. Typically, CVD
diamond forms in a very narrow temperature window. Deposition occurs when
the methane plus hydrogen mixture is activated. Energy to activate the process
may be in the form of radio frequency (RF), thermal (hot filament), microwave
plasma, high-voltage direct current (DC), and others.
The selection of an appropriate diamond deposition process depends on the
needs of the particular development program. Concerns about deposition rate,
hydrogen concentration, substrate compatibility, and the ability to coat three-
dimensional surfaces all should be taken into account. Three-dimensional CVD
diamond films have been made by hot filament assisted CVD. Obata and Mor-
imoto (1989) demonstrated the possibility of three-dimensional coatings by
forming diamond hemispheres and sleeves. Their work used a filament-type
reactor to produce these forms. Bachmann and Lydtin (1991) present a rudi-
mentary chart to help in the selection of a deposition process presented here as
Table 1. This type of chart should be viewed as a starting point from which the
fine points of the assorted deposition techniques can then be compared for such
things as cost, scalability, and ease of use as well as being capable of producing
the particular type of diamond that is desired.
Properties that make CVD diamond films excellent for one particular appli-
cation may be completely unsuitable for other uses. For example, the highest
thermally conductive CVD diamond film has the least desirable physical prop-
erties for use as a hydrostatic bearing coating. Good thermal conductivity results
from the material having a high degree of compositional purity and no nondia-
mond carbon in its structure. Nondiamond carbon has been found to help tough-
ness and resiliency in CVD films. A low defect density is also necessary for a
high thermal conductivity. Defects in the form of grain boundaries need to be