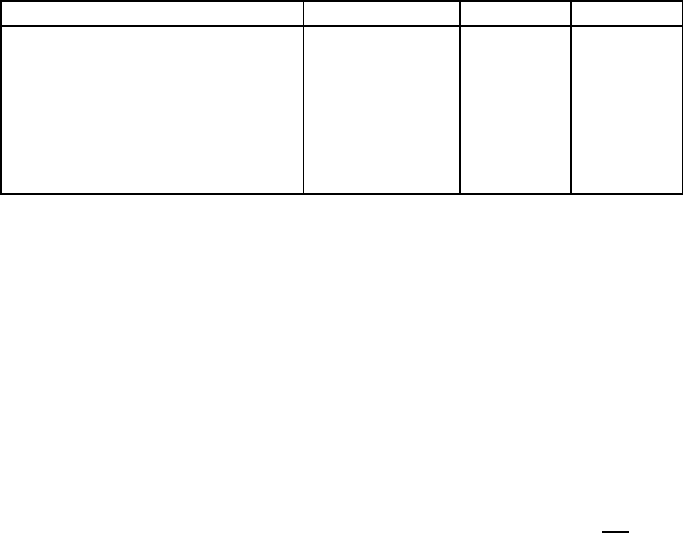
434 CHAPTER 5. PRACTICAL ASPECTS OF MAGNETIC METHODS
Table 5.33: Typical physical properties of ferrofluids, at 300 K.
Property Hydrocarbon Water Ester
Saturation polarization [T] 0.01-0.1 0.01-0.04 0.01-0.08
Density [kg/m
3
] 850-1250 1100-1400 1150-1400
Viscosity [Pa×s] 0.003-0.01 0.007-0.01 0.014-0.04
Boiling point [K] 350 299 422
Surface tension [10
3
N/m] 25-28 26 26
Thermal conductivity [W/m/K] 0.15 0.59
Electrical resistivity [m] 1.5×10
7
50-1000
Review of physical properties of ferrofluids
The chemical, mechanical and physical properties of ferrofluids correspond closely
to those of the carrier liquid. All commercially available ferrofluids utilizing or-
ganic carrier liquids are essentially electrically non-conductive. Their magnetic
saturation can be proportionately varied by dilution with the carrier fluid. Most
ferrofluids can be freeze-thawed without damage. Table 5.33 lists various phys-
ical properties of commercially available ferrofluids.
Selection of a magnetic fluid for material separation
When exposed to a non-homogeneous external magnetic field, a paramagnetic
liquid will exhibit an apparent density given by eq. (3.144):
d
=
i
+
0
j
EuE.
In order to achieve the apparent density equal, for instance, to the density of
diamond, that is 3500 kg/m
3
, in a saturated solution of manganese chloride (
i
= 1450 kg/m
3
, " =5.65×10
7
m
3
/kg and =8.2×10
4
(SI)), the product of
the magnetic induction and its gradient EuE must be equal to 30.8 T
2
/m. In a
magnet that can generate 2 T, the field gradient must be 15.4 T/m or 1.54×10
3
G/cm. This is a very high value indeed. In a superconducting magnet, at the
field induction of 20 T, the required field gradient is 1.54 T/m, or 154 G/cm.
The application of paramagnetic liquids is more feasible on a small laboratory
scale [P14] using, for example, a Frantz laboratory isodynamic separator. In
this separator, the product EuE can reach maximum value of 100 T
2
/m and
an apparent density of 10 000 kg/m
3
was achieved with maximum throughput
of the separator at 40 g/h and a maximum particle size of 1 mm [P14].
It is clear, therefore, that for separation in paramagnetic liquids very high
magnetic fields are required. This requirement results in high capital and run-
ning costs, and in the need to remove even very feebly magnetic material from
the feed. In addition, the working volume of such a separator would necessar-
ily be very small, which would limit the upper particle size, and its throughput
would be very low. Even in a massive and expensive installation, the throughput
would not exceed a few kilograms per hour.
These practical di!culties make separation in paramagnetic liquids (usually
termed magnetohydrostatic separation - MHS) an intriguing curiosity rather