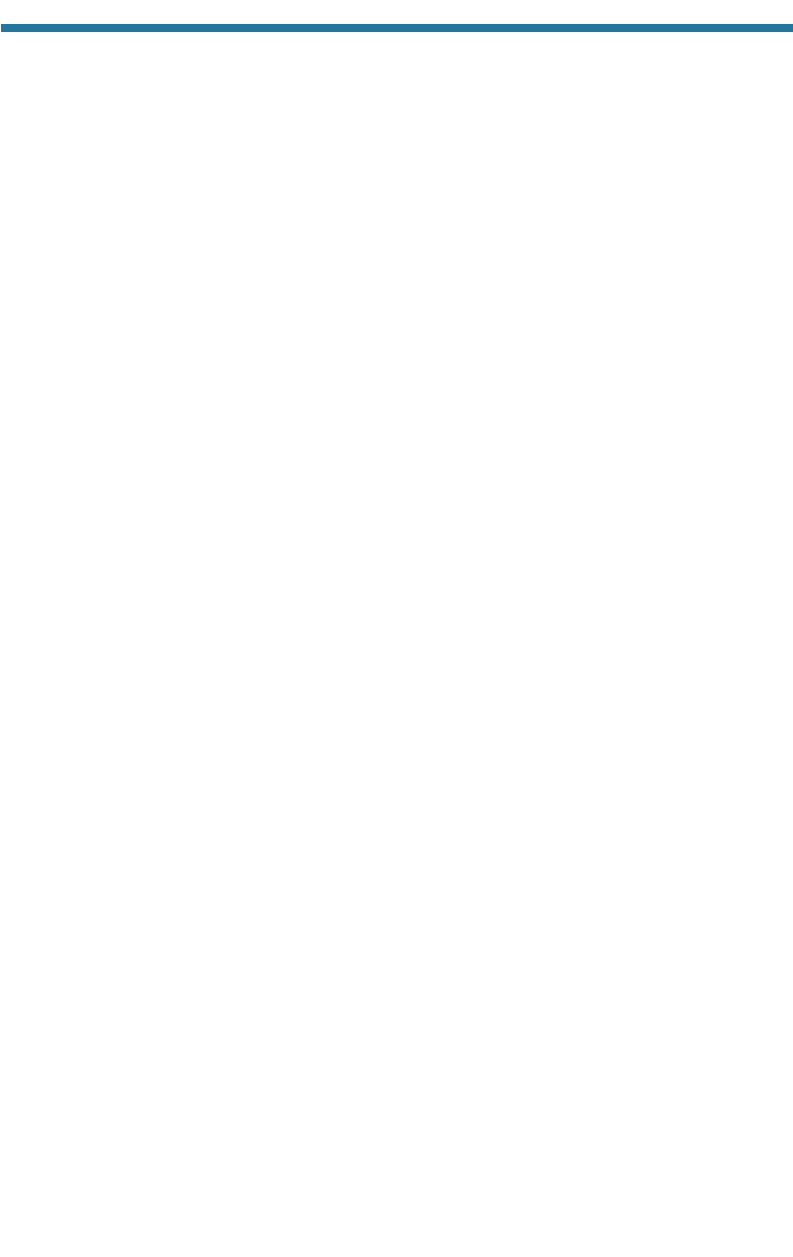
6.6.1. Vertical Pollutant Transport
Pressure systems affect vertical air motions and, therefore, pollutant dispersion by
forced and free convection (Section 3.2.2). In a semipermanent low-pressure system,
for example, near-surface winds converge and rise, dispersing near-surface pollutants
upward. In a semipermanent high-pressure system, winds aloft converge and sink, con-
fining near-surface pollutants. Both cases illustrate forced convection. In thermal
low-pressure systems, surface warming causes near-surface air to become buoyant and
rise. In thermal high-pressure systems, surface cooling causes near-surface air to
become negatively buoyant and sink or stagnate. Both cases illustrate free convection.
To understand better how free convection in thermal pressure systems and forced con-
vection in semipermanent pressure systems af
fect pollutant dispersion, it is necessary
to discuss adiabatic processes and atmospheric stability.
6.6.1.1. Adiabatic and Environmental Lapse Rates
Whether air rises or sinks buoyantly in a thermal pressure system depends on
atmospheric stability, which depends on adiabatic and environmental lapse rates.
These terms are discussed next.
Imagine a balloon filled with air. The air pressure inside the balloon exactly equals
that outside the balloon. Imagine also that no energy (such as solar or thermal-IR ener-
gy or latent heat energy created by condensation of water vapor) can enter or leave the
balloon, but that the balloon’s membrane is flexible enough for it to expand and con-
tract due to changes in air pressure outside the balloon. Suppose now that the balloon
rises. Because air pressure always decreases with increasing altitude, the balloon must
rise into decreasing air pressure. For the air pressure inside the balloon to decrease to
the air pressure outside the balloon, the balloon must expand in volume. This type of
expansion, caused by a change in air pressure alone, is called an adiabatic expansion.
Solar heating and latent heat release are diabatic heating processes and do not con-
tribute to an adiabatic expansion.
During an adiabatic expansion, kinetic energy of air molecules is converted to
work to expand the air. Because temperature is proportional to the kinetic energy of air
molecules (Equation 3.1), an adiabatic expansion cools the air. In sum, rising air
expands and expanding air cools; thus, rising air cools during an adiabatic expansion.
The rate of cooling during an adiabatic expansion near the surface of the Earth is
approximately 9.8 K or C per kilometer increase in altitude. This rate is called the
dry or unsaturated adiabatic lapse rate (
d
). Lapse rates are opposite in sign to
changes in temperature with height; thus, a positive lapse rate indicates that tempera-
ture decreases with increasing height.
If the balloon in our example rises in air saturated with water vapor, the resulting
adiabatic expansion cools the air and decreases the saturation vapor pressure of water
in the balloon, causing the relative humidity to increase to more than 100 percent and
water vapor to condense to form cloud drops, releasing latent heat. The rate of temper-
ature increase with increasing height due to this latent heat release is typically 4 K
km
1
, but it increases to 8 K km
1
in the tropics. Subtracting this latent-heat release
rate from the dry adiabatic lapse rate gives a net lapse rate during cloud formation of
between 6 and 2 K km
1
. This rate is called the wet-, saturated-, or pseudoadiabatic
lapse rate (
w
). It is the negative rate of change of temperature with increasing alti-
tude during an adiabatic expansion in which condensation also occurs. The wet
adiabatic lapse rate is applicable only in clouds.
EFFECTS OF METEOROLOGY ON AIR POLLUTION 157