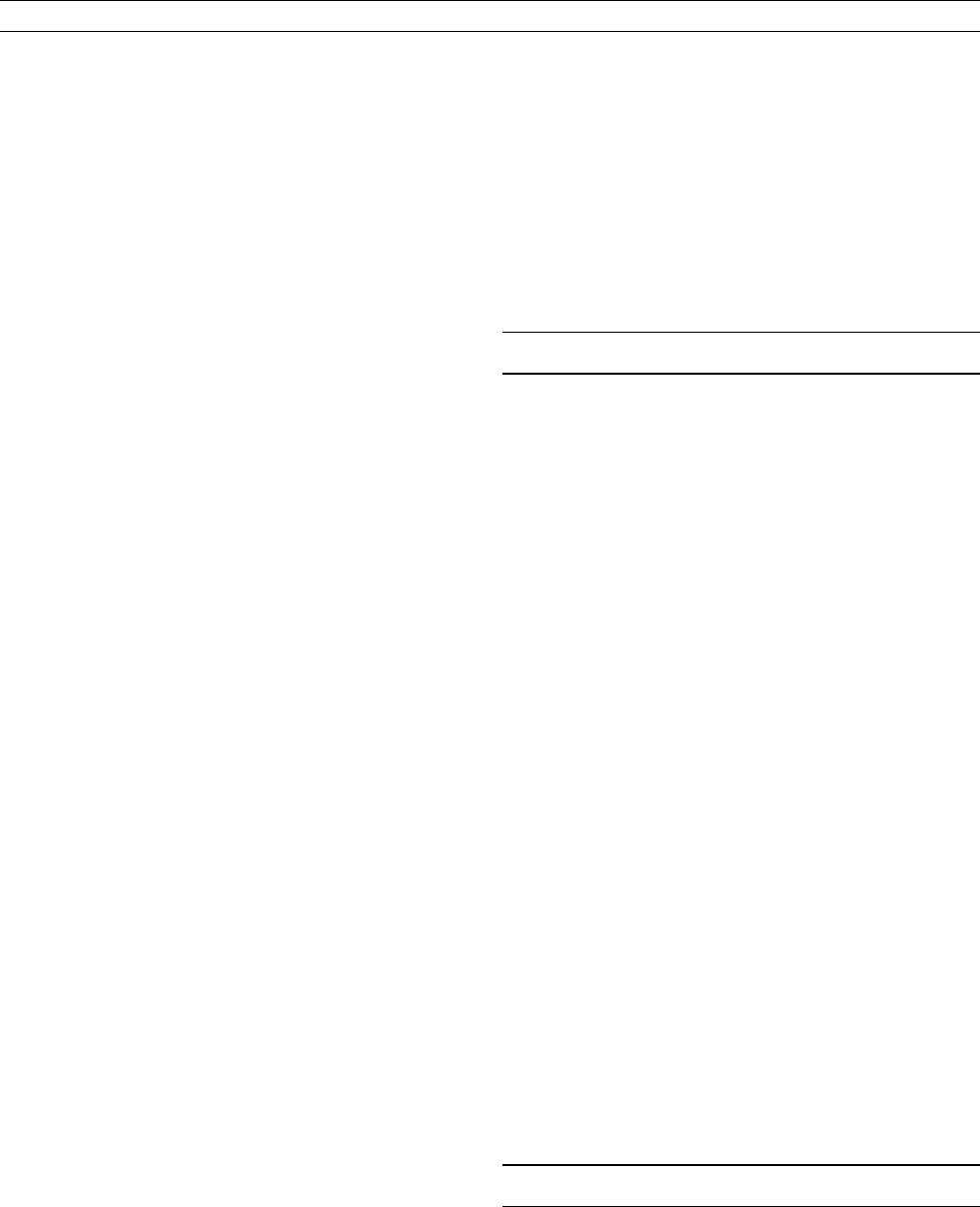
for airborne surveys is the diurnal variation, which generally has an
amplitude of tens of nanotesla. Shorter time-period variations due to
magnetic storms can be much larger (hundreds of nanotesla) and
severe enough to prevent survey data collection. Data can also be
degraded my micropulsations with short periods and amplitudes of
several nanotesla. Monitoring of the Earth’s field is an essential com-
ponent of a survey in order to mitigate these time-varying effects. One
or more base station magnetometers is used to track changes in the
field during survey operations. When variations are unacceptably
large, surveying is suspended and any flightlines recording during dis-
turbed periods are reflown. The smoothly changing diurnal variation
is removed from the data using tie-line leveling. Simply subtracting
this variation from the measured data is not sufficient since diurnal
changes may vary significantly over the survey area. Nonetheless,
the recorded diurnal can be used as a guide in the leveling process.
Tie-line leveling is based on the differences in the measured field at the
intersection of flightlines and tie-lines. If the distance and hence the
time taken to fly between these intersection points is small enough
then it can be assumed that the diurnal varies approximately linearly
and can be corrected for (Luyendyk, 1997).
Data display and interpretation
The final product resulting from an aeromagnetic survey is a set of
leveled flightline data that are interpolated onto a regular grid of mag-
netic field intensity values covering the survey region. These values
can be displayed in a variety of ways, the most common being a color
map or image, where the magnetic field values, based on their magni-
tude, are assigned a specific color. Similarly, the values can be repre-
sented as a simple line contour map. Both kinds of representation
can be used in a qualitative fashion to divide the survey area into sub-
regions of high and low magnetizations. Since the data are available
digitally, it is straightforward to use computer-based algorithms to
modify and enhance the magnetic field image for the specific purpose
of the survey. Transformation and filtering allows certain attributes of
the data to be enhanced, such as the effects due to magnetic sources
at shallow or deep levels, or occurring along a specified strike direc-
tion. More sophisticated methods may estimate the depths, locations,
attitudes, and the magnetic properties of magnetic sources.
Mark Pilkington
Bibliography
Gibson, R.I., and Millegan, P.S. (eds.), 1998. Geologic Applications of
Gravity and Magnetics: Case Histories. Tulsa, OK, U.S.A.: Society
of Exploration Geophysicists and American Association of Petro-
leum Geologists.
Grant, F.S., 1985. Aeromagnetics, geology and ore environments,
I. Magnetite in igneous, sedimentary and metamorphic rocks: An
overview. Geoexploration, 23: 303–333.
Hanna, W.F., 1990. Some historical notes on early magnetic surveying
in the U.S. Geological Survey. In Hanna, W.F., (ed.), Geologic
Applications of Modern Aeromagnetic Surveys. United States Geo-
logical Survey Bulletin 1924, pp. 63–73.
Hogg, R.L.S., 1989. Recent advances in high sensitivity and high reso-
lution aeromagnetics. In Garland, G.D. (ed.), Proceedings of
Exploration ’87, Third Decennial International Conference on
Geophysical and Geochemical Exploration for Minerals and
Groundwater. Ontario Geological Survey, Special Volume 3, pp.
153–169.
Horsfall, K.R., 1997. Airborne magnetic and gamma-ray data acquisi-
tion. Australian Geological Survey Organization Journal of Aus-
tralian Geology and Geophysics, 17:23–30.
Luyendyk, A.P.J., 1997. Processing of airborne magnetic data. Austra-
lian Geological Survey Organization Journal of Australian Geol-
ogy and Geophysics, 17:31–38.
Muffly, G., 1946. The airborne magnetometer. Geophysics, 11:321–334.
Reid, A.B., 1980. Aeromagnetic surveydesign.Geophysics,45:973–976.
Cross-references
Compass
Crustal Magnetic Field
Depth to Curie Temperature
IGRF, International Geomagnetic Reference Field
Magnetic Anomalies for Geology and Resources
Magnetic Anomalies, Modeling
AGRICOLA, GEORGIUS (1494–1555)
Agricola, which seems to have been the classical academic pen-name
for Georg Bauer, was born at Glauchau, Germany, on March 24, 1494
as the son of a dyer and draper. He studied at Leipzig University and
trained as a doctor, and became Town Physician to the Saxon mining
community of Chemnitz. His significance derives from his great treatise
on metal mining, De Re Metallica (1556), or “On Things of Metal.” This
sumptuously illustrated work was a masterly study of all aspects of
mining, including geology, stratigraphy, pictures of mineshafts, machin-
ery, smelting foundries, and even the diseases suffered by miners.
Book III of De Re Metallica contains a description and details of
how to use the miner’s compass which, by 1556, seems to have been
a well-established technical aid to the industry. Agricola drew a parallel
between the miner’s and the mariner’s compass, saying that the direction
cards of both were divided into equidistant divisions in accordance with
a system of “winds.” Agricola’s compass is divided into four quadrants,
with each quadrant subdivided into six: 24 equidistant divisions around
the circle. And just as a mariner named the “quarters” of his compass
from the prevailing winds, such as “Septentrio” for north and “Auster”
for south, so the miner likewise named his underground metal veins,
depending on the directions in which they ran.
Agricola also tells us that when prospecting for ore, a mining engi-
neer would use his compass to detect the veins of metal underground
and use it to plot their direction and the likely location of their strata
across the landscape.
Agricola, it was said, always enjoyed robust health and vigor. He
served as Burgomaster of Chemnitz on several occasions and worked
tirelessly for the sick during the Bubonic Plague epidemic that ravaged
Saxony during 1552–1553. Then, on November 21, 1555, he suddenly
died from a “four days” fever.
Allan Chapman
Bibliography
Agricola, G., De Re Metallica (Basilae, 1556), translated by Hoover,
H.C., and Hoover, L.H. (1912; Dover Edition, New York, 1950).
ALFVE
´
N WAVES
Introduction and historical details
Alfvén waves are transverse magnetic tension waves that travel along
magnetic field lines and can be excited in any electrically conducting
fluid permeated by a magnetic field. Hannes Alfvén (q.v.) deduced their
existence from the equations of electromagnetism and hydrodynamics
(Alfvén, 1942). Experimental confirmation of his prediction was found
seven years later in studies of waves in liquid mercury (Lundquist,
1949). Alfvén waves are now known to be an important mechanism
AGRICOLA, GEORGIUS (1494–1555) 3