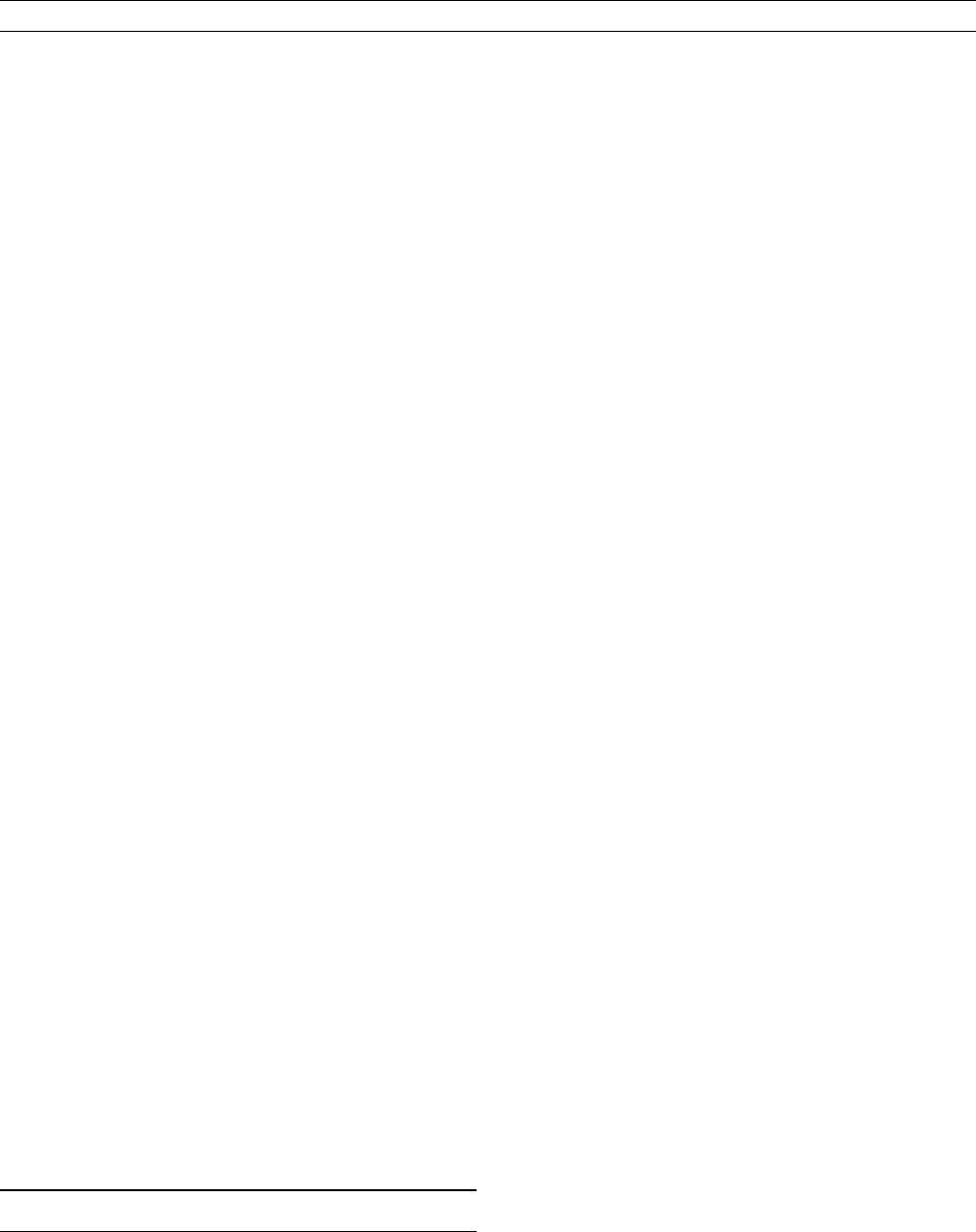
Bibliography
Doornbos, D.J., Spiliopoulos, S., and Stacey, F.D., 1986. Seismologi-
cal properties of D
00
and the structure of a thermal boundary layer.
Physics of the Earth and Planetary Interiors, 57: 225–239.
Garnero, E.J., Maupin, V., and Fouch, M.J., 2004. Variable azimuthal
anisotropy in the Earth’s lowermost mantle. Science, 306(5694):
259–261.
Karato, S. and Wu. P., 1993. Rheology of the upper mantle: A synth-
esis. Science, 260: 771–778.
Kendall J-M. and Silver, P.G., 1998. Investigating causes of D
00
aniso-
tropy. In Gurnis, M., Wysession, M.E., Knittle, E., and Buffett, B.
A. (eds.), The Core-Mantle Boundary Region. Geodynamic Series,
28, Washington, D.C.: American Geophysical Union, pp. 97–118.
Lay, T., Williams, Q., Garnero, E.J., Kellogg, L., and Wysession, M.E.,
1998. Seismic wave anisotropy in the D
00
region and its implications.
In Gurnis, M., Wysession, M.E., Knittle, E., and Buffett, B.A. (eds.),
The Core-Mantle Boundary Region. Geodynamic Series, 28,
Washington, D.C.: American Geophysical Union, pp. 299–318.
Mainprice, D., Barruol, G., and Ben Ismail, W., 2000. The seismic ani-
sotropy of the Earth’s mantle: From single crystal to polycrystal.
In Karato, S., Forte, A.M., Liebermann, R.C., Masters, T.G., and
Stixrude, L. (eds.), Earth’s Deep Interior: Mineral Physics and Tomo-
graphy from the Atomic to the Global Scale. Geophysical Monograph,
117, Washington, D.C.: American Geophysical Union, pp. 237–264.
Murakami, M., Hirose, K., Kawamura, K., Sata, N., and Ohishi, Y.,
2004. Post-perovskite phase transition in MgSiO
3
. Science, 304:
855–858.
Panning, M., and Romanowicz, B., 2004. Inferences of flow at the
base of the Earth’s mantle based on seismic anisotropy. Science,
303: 351–353.
Savage, M., 1999. Seismic anisotropy and mantle deformation: What
have we learned from shear-wave splitting? Reviews in Geophy-
sics, 37:65–106.
Silver, P.G., 1996. Seismic anisotropy beneath continents: Probing the
depths of geology. Annual Review Earth Space Sciences, 24:
385–432.
Sitxrude, L., 1998. Elastic constants and anisotropy of MgSiO
3
perovskite, periclase, and SiO
2
at high pressures. In Gurnis, M.,
Wysession, M.E., Knittle, E., and Buffett, B.A., (eds.), The Core-
Mantle Boundary Region. Geodynamic Series, 28, Washington
D.C.: American Geophysical Union, pp. 83–96.
Cross-references
Core-Mantle Boundary
Core-Mantle Boundary, Heat Flow Across
Core-Mantle Coupling, Electromagnetic
Core-Mantle Coupling, Thermal
Core-Mantle Coupling, Topographic
D
00
as a Boundary Layer
D
00
, Composition
D
00
, Seismic Properties
Seismic Phases
ULVZ, Ultralow Velocity Zone
D
00
, COMPOSITION
Both the mantle and the core of the Earth are fundamentally affected
by the properties of the material at the base of the mantle. This region
controls the heat flow between the core and the mantle; it may be the
source region for mantle plumes; and it is the lowermost window
through which the magnetic field of the Earth must propagate to reach
the surface. That the base of the mantle differs seismically from the
overlying mantle material was recognized nearly a century ago by
Gutenberg (1913). The D
00
zone was originally defined as a distinct
zone by Bullen (1949) as the layer with anomalously low increases
in seismic velocity with depth in the lowermost several hundred of
kilometers of the mantle. Since the importance of mantle convection
was recognized, the chemical composition of this lowermost 300 km
of Earth’s 2900 km-thick mantle has widely been viewed as approxi-
mately equivalent to that of the overlying lower mantle, with its seismic
properties perturbed by a large increase in temperature distributed over
the lowermost few hundred kilometers of the mantle. The superadia-
batic gradient associated with the basal boundary layer of the Earth’s
convecting mantle likely involves a gradual temperature increase of
1000 K to perhaps as much as 2000 K (if the layer is internally stra-
tified) across the D
00
zone. Such shifts in temperature can produce
decreased gradients of seismic velocities across this zone, a result that
generally agrees with laterally averaged seismic models of Earth’s
mantle, such as the Preliminary Reference Earth Model (PREM:
Dziewonski and Anderson, 1981).
However, improvements in seismic observations have driven a reas-
sessment of the possible roles of chemical differentiation and chemical
heterogeneity within D
00
. The specific seismic observations include:
a sporadic seismic discontinuity involving an increase of 1–3%
in shear and perhaps in compressional velocity at heights usually
200–300 km above the core-mantle boundary (CMB); a laterally het-
erogeneous zone 5–40 km above the CMB with decreases in compres-
sional and shear velocity of the order of 10% and 30%, respectively
(the ultralow velocity zone, or ULVZ); two large-scale slow structures
beneath Africa and the western Pacific spanning lateral dimensions of
2000 km and extending to heights of 1000 km above the CMB,
with shear decrements of 3% or larger; and pervasive and laterally
varying anisotropy within the D
00
layer (see Gurnis et al., 1998; Lay
et al., 1998; and D
00
, seismic properties, and D
00
, anisotropy). The che-
mical characteristics of D
00
have thus emerged as a multidisciplinary
problem, incorporating the anomalous seismic characteristics of this
layer, the somewhat ill-constrained properties of Earth materials at
the extreme conditions of the CMB (pressure of 135 GPa and tempera-
ture of 3500–5000 K), and the effects of changes in chemistry on the
electromagnetic properties of the deep mantle, and thus on the geo-
magnetic field.
The seismic observations of structural complexity within D
00
have
driven a broad range of proposals of possible chemical changes that
might be present within this region. These possibilities include: descent
of melts from the overlying mantle, enriching this zone in incompatible
and volatile elements; interaction of material with the underlying core,
producing iron enrichment, and contributions from subducted slab
material. Additionally, a different chemistry of this region might have
been created early in the history of the planet, through early differentia-
tion processes or perhaps even during planetary accretion. All such pro-
posed changes in chemistry must not dramatically alter the seismic
velocity or density in this region from that within the lowermost
mantle: seismic travel-time and normal mode constraints show that
the bulk of D
00
can only differ in velocity and density by a few percent
from that within the deep mantle (see Earth structure, major divisions).
This constraint is, however, relaxed in the lowermost 5–40 km of the
mantle, where dramatic changes of seismic wave velocities have been
observed.
From a geomagnetic perspective, the importance of the chemistry of
the lowermost mantle lies primarily in its effect on the propagation
of Earth’s magnetic field through the basal layer of the mantle. If the
electrical conductivity of the lowermost mantle is markedly elevated
from that of the overlying material, through enrichment in iron, the
presence of partial melt, or both, then temporal variations of the mag-
netic field could be attenuated within such a layer (see Mantle, electri-
cal conductivity, mineralogy). Moreover, any electromagnetic coupling
between the core and mantle would generate a torque on the overlying
mantle: such a torque has been associated with shifts in the nutation of
the Earth and changes in the length of day (e.g., Buffett, 1992). If high
conductivity zones were heterogeneously distributed near the base of
D
00
, COMPOSITION 149