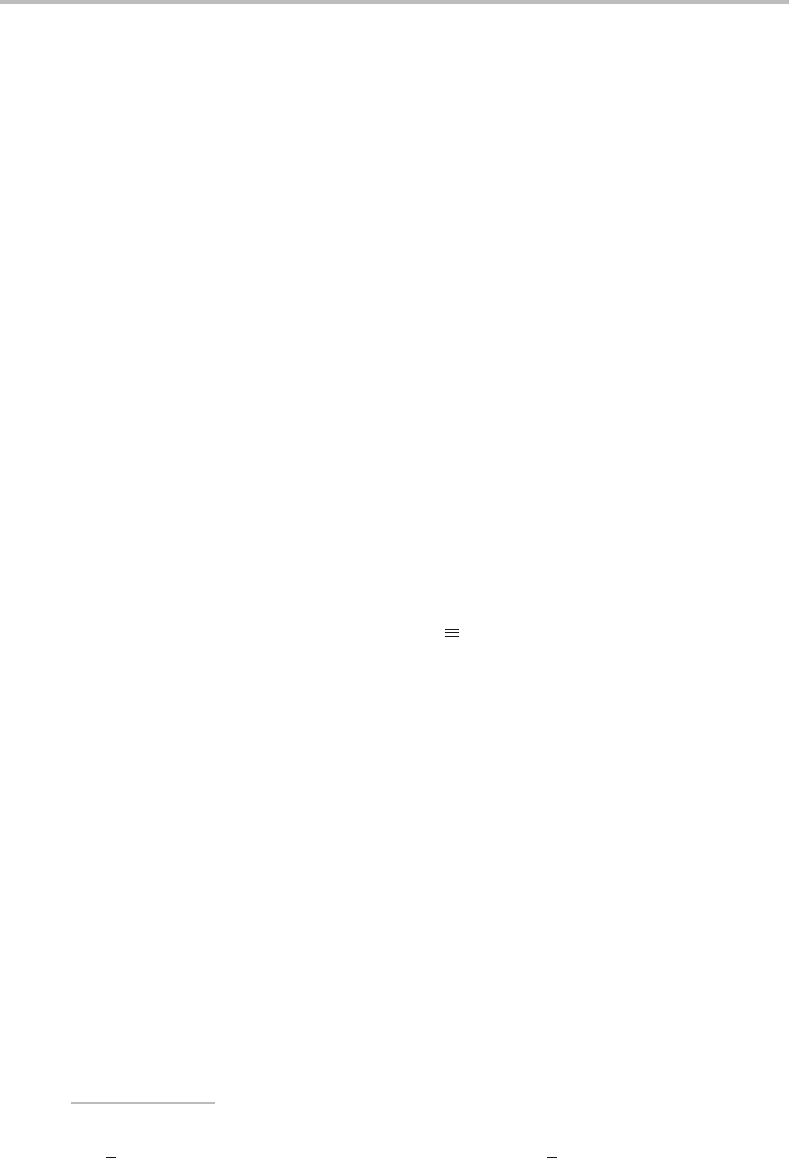
172 Case Studies
21.1 Experimental details
Al(H
2
O) is unlikely to be the sole product when laser ablating solid aluminium in the
presence of H
2
Ovapour. Despite the effort to cool the gas mixture, other reactions are almost
certainly unavoidable. Furthermore, a variety of clusters and complexes might be formed
involving multiple metal atoms and/or multiple water molecules. Experimental conditions
can be optimized to favour production of Al(H
2
O), e.g. by adjusting the partial pressure
of water vapour, but there will always be other species in the supersonic jet. Consequently,
some means of selectively detecting the spectrum of Al(H
2
O) is beneficial.
Agreiter and co-workers recorded spectra using the PFI version of ZEKE, in which the
laser wavelength is tuned to just below the ionization threshold and the complex is then
ionized by application of a delayed pulsed electric field (see Section 12.6 for more details).
The apparatus employed by Agreiter et al. was also equipped with a time-of-flight mass
spectrometer, and so it proved possible to estimate the ionization energies of Al(H
2
O)
n
complexes with different n by tuning the laser wavelength and looking for the onset of
photoionization in a given mass channel. In this way, Agreiter et al. were able to confirm
earlier work by Misaizu and co-workers in which the ionization energies of Al(H
2
O)
n
complexes were found to decrease rapidly as a function of n [2]. Since pulsed field ionization
is only observed close to the threshold for ionization, this information provides the means
of distinguishing between the spectra of the various possible Al(H
2
O)
n
complexes. The
ionization energy of Al(H
2
O) is approximately 5.1 eV, much lower than that expected for
chemical products such as HAlOH. It is therefore possible to be confident that the ZEKE
spectra recorded in the region close to 5.1 eV (
243 nm) originate from Al(H
2
O).
21.2 Assignment of the vibrationally resolved spectrum
ZEKE spectra of Al(H
2
O) and Al(D
2
O) are shown in Figure 21.1. Both spectra show an
obvious vibrational progression. In addition, there is finer structure, which is particularly
noticeable in the case of Al(D
2
O). This additional structure will be discussed later.
It is worth briefly reviewing what ZEKE spectroscopy reveals. In essence, resonant
transitions between energy levels of the neutral molecule and the ion are recorded. Conse-
quently, assuming most of the Al(H
2
O) and Al(D
2
O) complexes are initially in the zero-
point vibrational level, as is reasonable given that they are entrained in a supersonic jet,
then the observed vibrational structure is representative of the corresponding cations.
The fact that a single vibrational progression dominates the spectrum makes the assign-
ment relatively easy. The active mode has a frequency of approximately 328 cm
−1
for
Al
+
(H
2
O), as deduced from the spacing between adjacent peaks in the progression. The
only challenge is to identify the specific vibrational mode responsible. Al(H
2
O) and its
cation each have six degrees of vibrational freedom, which are illustrated in Figure 21.2.
1
1
The form of the six vibrations can be readily deduced. H
2
O will contribute the same three vibrational modes as the
free H
2
O molecule, although the mode frequencies will differ from those of the free molecule. The formation of
an Al
O bond will then add three further vibrations, an intermolecular (Al O) stretching mode and two bending
modes, one an in-plane and the other an out-of-plane (umbrella-like) deformation.