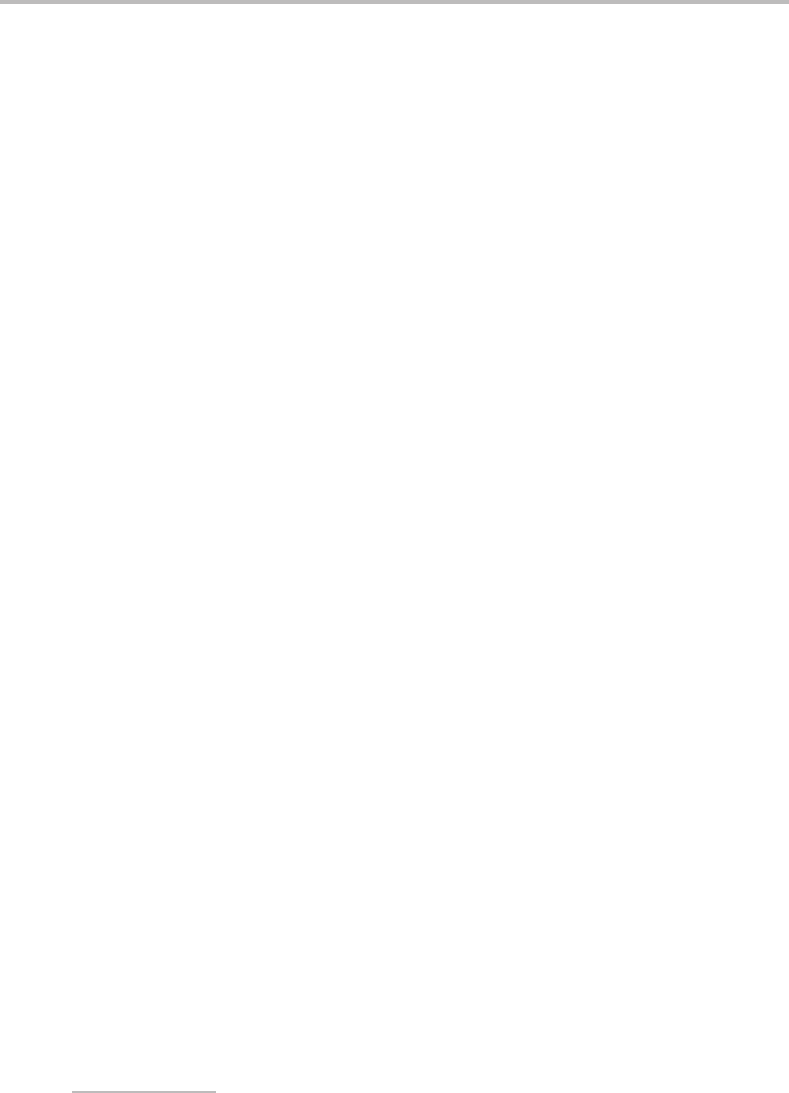
12 Photoelectron spectroscopy
103
In VUV noble gas resonance lamps, a high voltage DC discharge along a capillary tube
1
is
employed to drive noble gas atoms up to excited electronic states. The electronic transition
back to the ground state is then responsible for the radiation. For helium, the principal
emission line is at 21.218 eV (λ = 58.4 nm) and arises from the transition
1
P(1s
1
2p
1
) →
1
S(1s
2
). This line is referred to as the HeIα line, the I signifying emission from neutral
helium and the α designating that this is the first of a series of possible np → 1s transitions.
Other transitions do occur, not only from neutral helium but also He
+
(these are labelled
HeII transitions), but they are normally much weaker than the HeIα line. Other gases can
be used. For example, neon gives two NeI lines, one at 16.671 and the other at 16.848 eV.
However, helium is the most commonly used both because it is cheaper than neon and
because the higher photon energy means that the valence orbitals of most molecules can be
photoionized with the HeIα line.
The electron kinetic energy spectrum is obtained by passing the ejected electrons through
an energy analyser. This analyser is based on an electric or magnetic field, usually the former,
to distinguish the electrons according to their kinetic energies. There are two main analyser
types, retarding field and deflection analysers.
Retarding field devices transmit only those electrons that have energies higher than the
retarding potential, and to obtain a spectrum the retarding potential is scanned. This type
of analyser is rarely used nowadays and we shall discuss it no further.
Deflection analysers, as the name implies, separate electrons by forcing them to follow
different paths according to their velocities. There are a number of different types, including
the parallel plate analyser (this uses an electric field applied between two parallel plates)
and the cylindrical mirror analyser (containing two charged coaxial cylinders). However,
the only one that we will discuss in any detail is the hemispherical analyser, since it is simple
to understand and is widely used.
The basic geometry of the hemispherical analyser is illustrated in the overall schematic of
a photoelectron spectrometer in Figure 12.1. The name derives from the use of two concentric
hemispherical electrodes, both charged to a potential with the same magnitude but opposite
signs; the inner one is positive and the outer negative. The entrance and exit to the analyser
are restricted by slits that define the range of acceptable entrance and exit trajectories of the
electrons. Electrons that pass through the entrance slits after photoionization may traverse
the analyser and out through the exit slits only by following a specific curved path, but
they will do so only if they have the correct energy (determined by the selected voltages
on the hemispheres). The fate of electrons with higher or lower kinetic energies is clear
from the figure; the electric field is either too weak or too strong, respectively, to allow them
to follow the correct trajectory and they are lost in collisions with the walls. An electron
kinetic energy spectrum is obtained by measuring the electron current at the detector as a
function of the voltage applied to the hemispheres. The voltage can be used to calculate the
electron kinetic energy.
2
1
The capillary serves two purposes. First it helps to collimate the radiation. Second, it helps to minimize the amount
of sample gas passing into the discharge region, since there are no suitable window materials for wavelengths
shorter than 100 nm.
2
In practice one cannot extract a particularly accurate electron kinetic energy by calculations based solely on the
applied voltage. This is because the electron energy also depends on the local charges on any surfaces it passes,