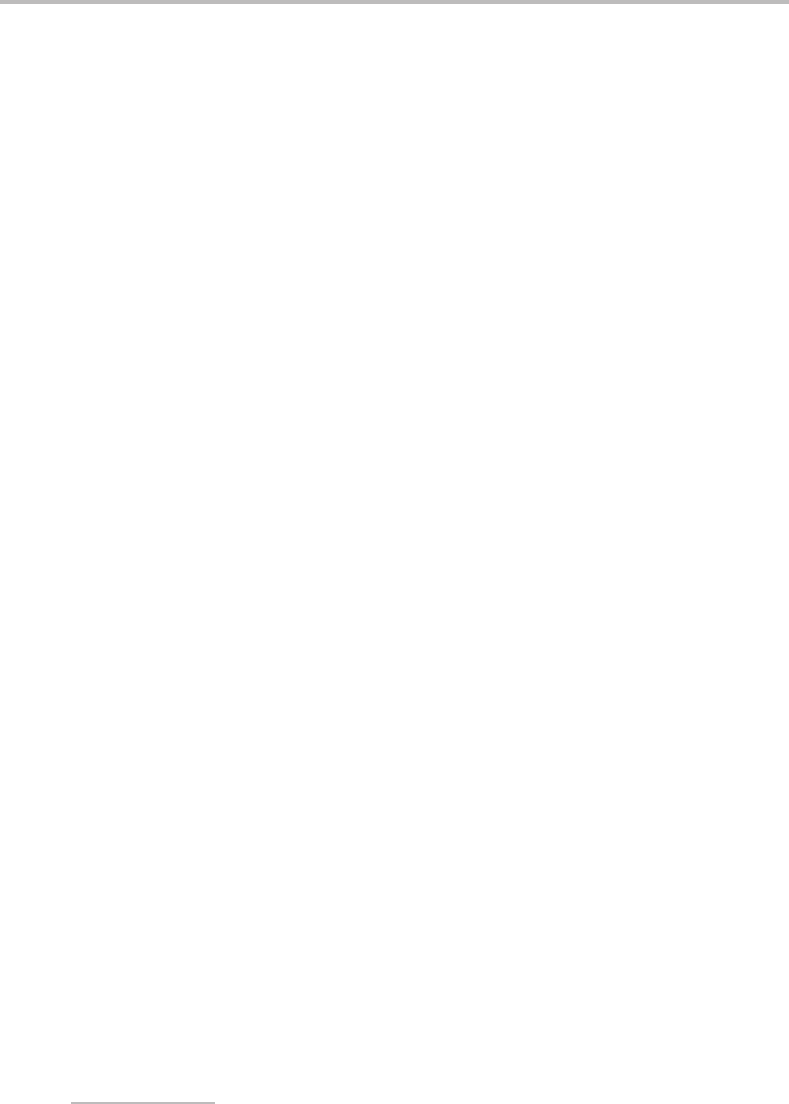
72 Experimental techniques
can collisionally stabilize the complex before it falls apart (cf. the need for a third body in
the recombination reactions of free radicals, such as CH
3
+ CH
3
+ M → C
2
H
6
+ M).
4
The number of two-body collisions downstream of the nozzle is proportional to P
r
D,
where P
r
is the pressure in the gas reservoir behind the nozzle and D is the diameter of the
orifice. The three-body collision rate depends on P
2
r
D, and so complex formation is favoured
by high reservoir pressures. This idea has been widely exploited by gas phase spectroscopists
to study van der Waals complexes. For example, the addition of noble gas atoms to simple
species, such as metal atoms or small molecules, or onto larger molecules such as benzene,
tetrazine, and azulene, has been achieved. The study of complexes involving inert gas atoms
is important because it provides detailed information on van der Waals forces, and the low
temperature environment in a supersonic jet is excellent for studying these very weakly
bound species. Other types of complexes, such as hydrogen-bonded dimers and trimers,
have also been prepared in the gas phase by this means [1].
Many other fascinating species can be formed in supersonic jets. For example, free
radicals may be produced by photolysis. The usual method is to cross the jet with an
ultraviolet laser beam close to the nozzle so that subsequent cooling in the expanding gas
is possible. Many different free radicals have been investigated by this route, ranging from
simple diatomic and triatomic species, such as CH, CH
2
, HCO, OH, to larger radicals such as
cyclopentadienyl (C
5
H
5
)[2]. The simplification of the spectra of these molecules brought
about by supersonic expansion has led to remarkable advances in our knowledge of the
structures and properties of these important chemical intermediates.
Molecular ions can also be studied in supersonic jets. One way to make these is by
use of an electrical discharge (which can also be used to make free radicals). A possible
arrangement for a discharge/supersonic jet experiment is shown in Figure 8.3.
Finally, it is also possible to make highly reactive molecules in the region just upstream
of the nozzle, i.e. just prior to expansion, and then entrain these molecules in a supersonic
jet. This idea has been widely exploited, most notably in the production of metal-containing
molecules. Metal atoms can be ablated from metal surfaces using high intensity pulsed
lasers, such as Nd:YAG or excimer lasers (see Chapter 10), and can then be carried to the
point of expansion by a suitable carrier gas. If a reagent is seeded into the inert carrier gas,
other species can be made by chemical reactions, such as metal hydrides, metal carbides,
metal halides, and organometallics.
8.3 Matrix isolation
Inert solid hosts provide an alternative environment for investigating individual molecules.
The noble gas solids are the best examples since they are virtually chemically inert and have
no absorption bands in the infrared, visible, and near-UV regions. The basic idea is to mix
the molecules of interest with an excess of noble gas and this mixture is then condensed on
4
Examples of cluster formation through two-body collisions are known. In these cases, it is thought that the initial
two-body collision leads to the formation of a reasonably long-lived orbiting complex. Providing the lifetime of
this complex is sufficiently long, it can be stabilized by another two-body collision leading to the formation of a
stable cluster.