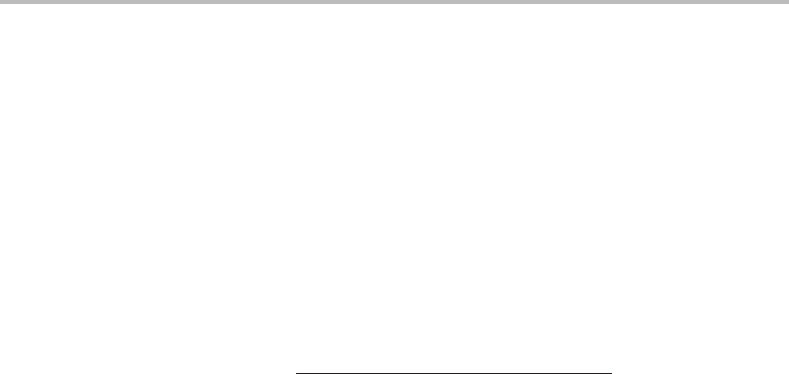
90 Experimental techniques
usually a PMT. PMTs have phenomenal sensitivities and are even capable of detecting single
photons in some cases. When the laser is off-resonance, no fluorescence will be produced,
and therefore the PMT registers no signal. However, at resonant wavelengths fluorescence
is possible and so absorption can be registered by detecting emission from the excited state.
This is the basic idea of laser excitation spectroscopy,inwhich a spectrum is obtained by
measuring the fluorescence intensity as a function of laser wavelength.
There are several important points to note about laser excitation spectroscopy. First, while
there is a clear similarity between laser excitation spectroscopy and absorption spectroscopy,
there is also an important difference. The intensity of peaks in a laser excitation spectrum
depends on both the absorbance of the sample and the fluorescence quantum yield of the
excited state. The fluorescence quantum yield is defined as
f
=
rate of photon emission by excited state
rate of photon absorption
(11.2)
A fluorescence quantum yield of unity implies that all molecules excited to the upper
electronic state relax via photon emission. However, competition from other decay routes
(see below) may not only lower
f
,but may also cause it to change from one excited state
level to another. As a result absorption and fluorescence excitation spectra may look very
different.
The high sensitivity of LIF spectroscopy arises from the low background signal received
by the PMT at off-resonance laser wavelengths. Even though any fluorescence produced
may be very small, it is easily detected by the PMT and therefore if the off-resonance
signal is much smaller still then an extremely high signal-to-noise ratio can be achieved. In
practice the off-resonance signal is never zero. The principal cause is scattered light from
the laser. This can be minimized by keeping potential scattering sites out of the path of the
laser. Furthermore, scattered laser light can be virtually eliminated if at least a portion of
the fluorescence is at longer wavelengths than the laser. If this condition is satisfied, and
it often is for many molecules, then an optical filter, which will only transmit wavelengths
longer than that of the laser, can be inserted in front of the PMT.
In laser excitation spectroscopy the fluorescence serves only as a means of detecting the
absorption process. However, the fluorescence itself clearly contains spectroscopic infor-
mation since it arises from emission to lower energy levels. If the emission is dispersed in
a monochromator, the spectrum obtained will be the emission spectrum originating from
a specific (laser-excited) upper state. This type of spectroscopy goes by several names,
including dispersed fluorescence spectroscopy, laser-excited emission spectroscopy, and
single vibronic level fluorescence spectroscopy;wewill use the first of these throughout
this text.
In laser excitationspectroscopythe resolution is often limited by the linewidth of the laser.
For pulsed dye lasers, linewidths of ∼0.03 cm
−1
can be obtained relatively straightforwardly.
If narrower linewidth lasers are used, such as CW dye lasers or specialized pulsed dye lasers,
other factors may begin to limit the resolution, such as Doppler broadening. If steps are taken
to minimize Doppler broadening, a resolution of better than 0.001 cm
−1
can be attained.
With such a high resolution, rotationally resolved electronic spectra of quite large molecules
can be tackled.