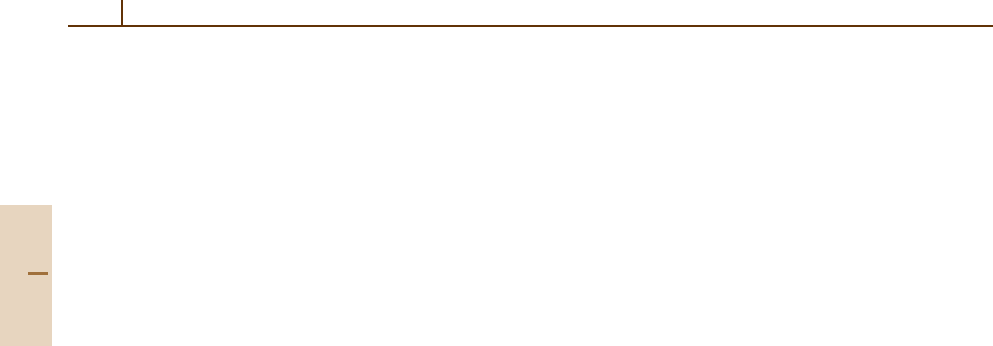
1242 Part G Applications
rich star surrounded by dust and gas it ejected in a strong
stellar wind. The central star is so shielded that it is
almost undetectable at optical wavelengths, and was not
discovered until the 2 µm survey. IRC 10216 is where
most of the circumstellar molecules are detected, and
has greatly increased our understanding of circumstellar
envelopes.
The envelopes are ejected by the red giant in its
final phase of evolution. The mass loss rates increase
to ∼ 10
−4
solar masses per year and temperatures in
the envelope are of order 1000 K. Close to the star, the
density is high and the chemistry is characteristic of
thermal equilibrium. The situation is quite unlike any
interstellar environments. For example in IRC 10216,
the HNC is over one hundred times less abundant than
HCN, whereas in molecular clouds, they have about the
same abundance.
If the star is oxygen-rich, large amounts of H
2
Oare
formed and if it is carbon-rich, C
2
H
2
.Thishightem-
perature environment forms both molecules and grains.
The high obscuration of the central source indicates that
grains are formed in these envelopes. Polycyclic aro-
matic hydrocarbons (PAH’s) or some similar species are
observed in carbon rich planetary nebulae. These large
molecules must have been produced when the object
was a carbon rich circumstellar envelope.
As the material flows out from the star the den-
sity and temperature decrease. As the density becomes
lower, three body reactions become less important and at
some point the products of these reactions are frozen out
in a similar manner to the evolution of molecules in the
early universe (Sect. 82.10). In the outermost portions of
the circumstellar envelope, molecules are dissociated by
interstellar UV photons. The penetrating UV radiation
is shielded by dust, H
2
, and CO. The relative abun-
dances can vary rapidly with radius, and observations
provide abundance and radial distribution, a wealth of
data for modelers. Circumstellar chemistry is reviewed
by Omont [82.32] and recent chemical models are given
in [82.33–35].
82.6 Supernova Ejecta
A supernova, the explosion of a massive star following
core collapse, is one of the most spectacular displays in
the Universe. The explosion occurs when the iron core
of a massive star collapses to form a neutron star and the
rebound shock and neutrino flux eject the outer portion
of the star. The ejected portions of the star are rich in
heavy elements produced in the interior of the progenitor
star.
We are fortunate to have had in our lifetime a super-
nova which was close and in an unobscured line of
sight. Supernova 1987A, the first supernova observed in
1987, went off in the Large Magellanic Cloud, a small
satellite galaxy to our own. It was the first supernova
visible to the naked eye in nearly 400 years (since
the Kepler supernova in 1604). Using the full range of
modern astronomical instruments has allowed us to get
detailed spectra of the evolving ejecta which has greatly
increased our knowledge of supernovae. We will use
SN1987A as an example of supernova ejecta.
Initially the temperature of the ejecta of SN1987A
was high, ≈ 10
6
K, but it quickly cooled through adi-
abatic expansion and radiation from the photosphere.
The temperature leveled off at several thousand degrees
because of heating by radioactive nuclei, first
56
Ni and
then
56
Co, formed in the explosion. The dynamics is
homologous free expansion: the velocity scales linearly
with the radius r(t) = vt where v the velocity and t the
time since the explosion.
The ejecta at first were optically thick and the
spectrum resembled that of a hot star continuum with
absorption lines from the surface. After a few days, the
temperature dropped, but the ejecta remained optically
thick and continued to show strong continuum emission.
As the ejecta expand, the temperature drops, the ejecta
become optically thin, and the spectrum is dominated by
strong emission lines, superficially resembling an emis-
sion nebula Sect. 82.2. The emission is dominated by
neutral atoms and singly ionized species.
The gas is heated and ionized by the gamma rays
from radioactive decay. The gamma rays Compton scat-
ter, producing X-rays and fast electrons. The X-rays
further ionize the gas and produce multiply charged ions
through the Auger process. These multiply charged ions
recombine through charge transfer with neutral atoms.
Further charge transfer determines the relativeionization
of different species, with the lowest ionization poten-
tial species more ionized than the higher ionization
potential species. The development of the infrared and
optical spectrum of Supernova 1987A has been recently
reviewed by McCray [82.36].
One of the great surprises in the spectrum of Super-
nova 1987A was the discovery of molecules in the
Part G 82.6