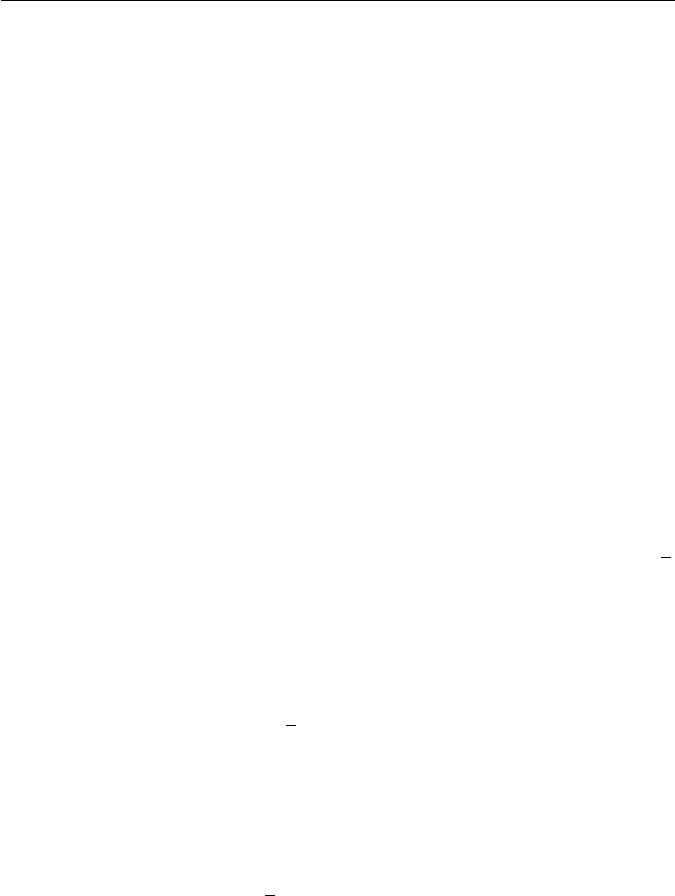
10.5 TWIP STEELS 229
MnO begins to cover the surface leading to a deterioration in the ability of the
liquid zinc to wet the surface. The aluminium-containing steels are therefore
better suited to continuous galvanizing lines.
It is clear that silicon and manganese must diffuse to the surface to form
oxides. Some of the diffusion flux is via grain boundaries. When phosphorus
is present, its segregation to the grain boundaries reduces the boundary
diffusion-flux, thereby reducing the extent to which oxides form. Such steels
are more amenable to wetting by molten zinc (in contrast, the same effect
makes it more difficult to form iron–zinc compounds during the galvannealing
process).
10.5 TWIP STEELS
There are three essential modes by which steels can be permanently deformed
at ambient temperature, without recourse to diffusion. Individual dislocations
whose Burgers vectors correspond to lattice vectors can glide, leading to a
change in shape without altering the crystal structure or volume. In contrast, a
displacive transformation (e.g. martensite or bainite) results not only in a plastic
strain, but also a change of crystal structure and density; this is the phenomenon
exploited in the TRIP steels.
The third mode of deformation is mechanical twinning, in which the crystal
structure of the steel is preserved but the twinned region is reoriented in the
process. Mechanical twinning results in a much larger shear strain s =1/
√
2,
compared with displacive transformations where s is typically 0.25. There is a
particular class of extraordinarily ductile alloys of iron, known as the TWIP
steels, which exploit mechanical twinning to achieve their properties.
TWIP stands for twinning-induced plasticity. The alloys are austenitic and
remain so during mechanical deformation, but the material is able to accommo-
date strain via both the glide of individual dislocations and through mechanical
twinning on the {111}
γ
< 11
2 >
γ
system. The alloys typically contain a
large amount of manganese, some aluminium and silicon (e.g. Fe–25Mn–3Si–
3Al wt%) with carbon and nitrogen present essentially as impurities. Larger
concentrations of carbon may be added to enhance strength. At high man-
ganese concentrations, there is a tendency for the austenite to transform into
ǫ-martensite (hexagonal close packed) during deformation. ǫ-martensite can
form by the dissociation of a perfect a/2 < 011>
γ
dislocation into Shockley
partials on a close packed {11
1}
γ
plane, with a fault between the partials. This
faulted regionrepresents a three layer thick plate of ǫ-martensite.A reduction in
the fault energy therefore favours the formation of this kind of martensite. The
addition of aluminium counters this because it raises the stacking fault energy
of the austenite. Silicon has the opposite effect of reducing the stacking fault
energy, but like aluminium, it leads to a reduction in the density of the steel;
the combination of Al and Si at the concentrations used typically reduces the
overall density from some 7.8 g cm
−3
to about 7.3 g cm
−3
.