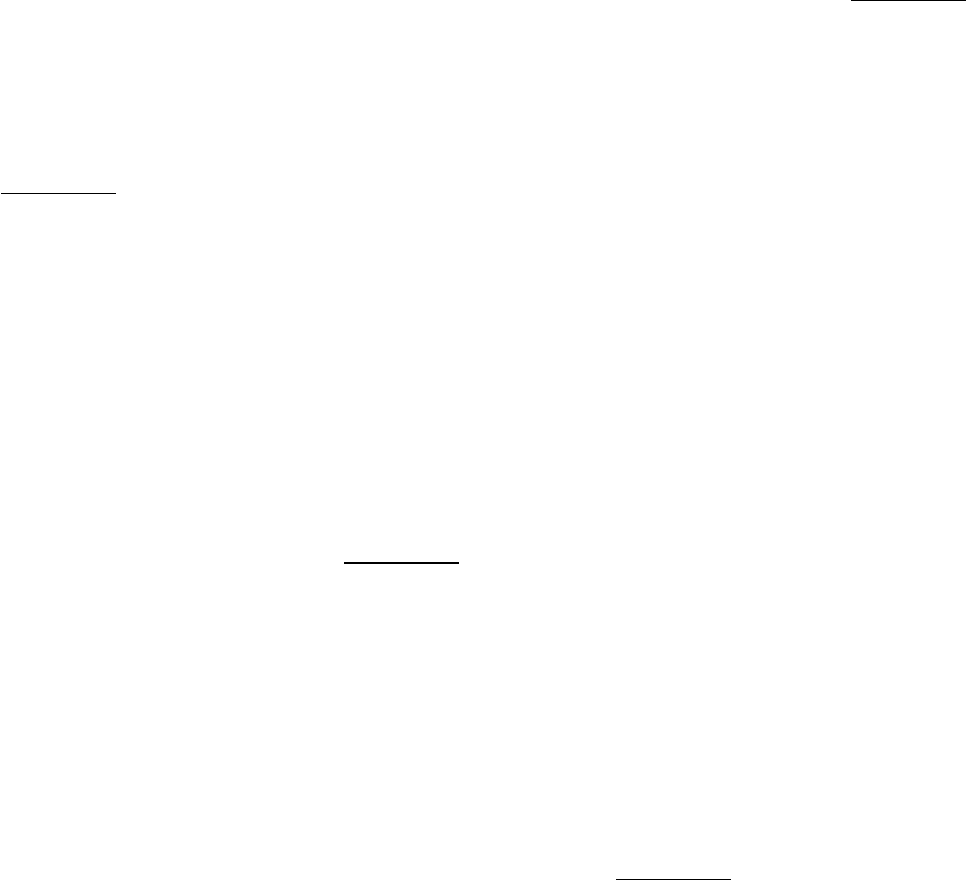
Notably, the nucleotide-binding site in actin corresponds to the ATP-binding site in hexokinase. Thus, actin evolved
from an enzyme that utilized ATP as a substrate.
More recently, a closer prokaryotic homolog of actin was characterized. This protein, called MreB, plays an important
role in determining cell shape in rod-shaped, filamentous, and helical bacteria. The internal structures formed by MreB
are suggestive of the actin cytoskeleton of eukaryotic cells, although they are far less extensive. Even though this protein
is only approximately 15% identical in sequence with actin, MreB folds into a very similar three-dimensional structure.
It also polymerizes into structures that are similar to F-actin in a number of ways, including the alignment of the
component monomers.
34.2.3. Motions of Single Motor Proteins Can Be Directly Observed
Muscle contraction is complex, requiring the action of many different myosin molecules. Studies of single myosin
molecules moving relative to actin filaments have been sources of deep insight into the mechanisms underlying muscle
contraction and other complex processes.
A powerful tool for these studies, called an optical trap, relies on highly focused laser beams (Figure 34.17). Small beads
can be caught in these traps and held in place in solution.
The position of the beads can be monitored with nanometer precision. James Spudich and coworkers designed an
experimental arrangement consisting of an actin filament that had a bead attached to each end. Each bead could be
caught in an optical trap (one at each end of the filament) and the actin filament pulled taut over a microscope slide
containing other beads that had been coated with fragments of myosin such as the heavy meromyosin fragment (see
Figure 34.17). On the addition of ATP, transient displacements of the actin filament were observed along its long axis.
The size of the displacement steps was fairly uniform with an average size of 11 nm.
The results of these studies, performed in the presence of varying concentrations of ATP, are interpreted as showing that
individual myosin heads bind the actin filament and undergo a conformational change (the power stroke) that pulls the
actin filament, leading to the displacement of the beads. After a period of time, the myosin head releases the actin, which
then snaps back into place.
34.2.4. Phosphate Release Triggers the Myosin Power Stroke
How does ATP hydrolysis drive the power stroke? A key observation is that the addition of ATP to a complex of myosin
and actin results in the dissociation of the complex. Thus, ATP binding and hydrolysis cannot be directly responsible for
the power stroke. We can combine this fact with the structural observations described earlier to construct a mechanism
for the motion of myosin along actin (Figure 34.18). Let us begin with myosin-ADP bound to actin. The release of ADP
and the binding of ATP to actin result in the dissociation of myosin from actin. As we saw earlier, the binding of ATP
with its γ-phosphate group to the myosin head leads to a significant conformational change, amplified by the lever arm.
This conformational change moves the myosin head along the actin filament by approximately 110 Å. The ATP in the
myosin is then hydrolyzed to ADP and P
i
, which remain bound to myosin. The myosin head can then bind to the surface
of actin, resulting in the dissociation of P
i
from the myosin. Phosphate release, in turn, leads to a conformational change
that increases the affinity of the myosin head for actin and allows the lever arm to move back to its initial position. The
conformational change associated with phosphate release corresponds to the power stroke. After the release of P
i
, the
myosin remains tightly bound to the actin and the cycle can begin again.
How does this cycle apply to muscle contraction? Myosin molecules self-assemble into thick bipolar structures with the
myosin heads protruding at both ends of a bare region in the center (Figure 34.19). Approximately 500 head domains
line the surface of each thick filament. These domains are paired in myosin dimers, but the two heads within each dimer
act independently. Actin filaments associate with each head-rich region, with the barbed ends of actin toward the Z-line.
In the presence of normal levels of ATP, most of the myosin heads are detached from actin. Each head can
independently hydrolyze ATP, bind to actin, release P
i
, and undergo its power stroke. Because few other heads are