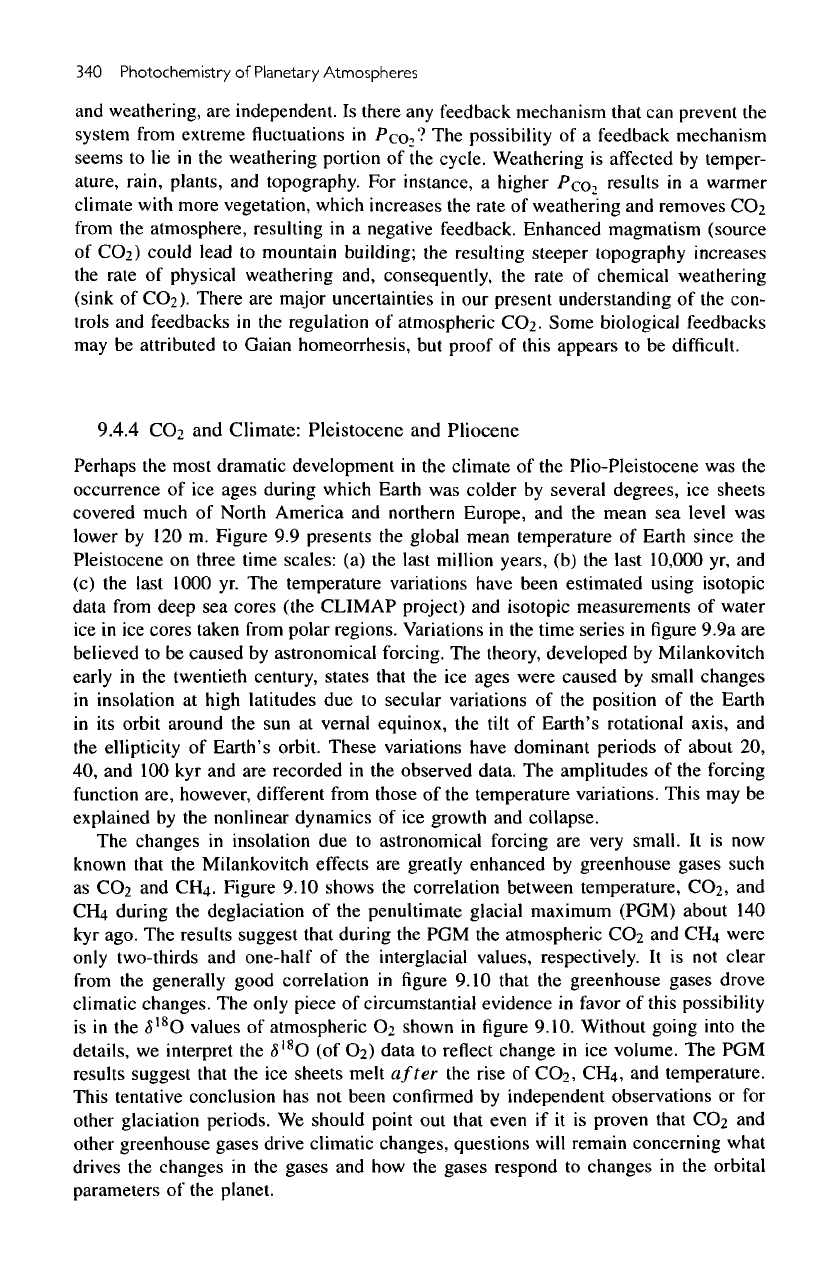
340
Photochemistry
of
Planetary
Atmospheres
and
weathering,
are
independent.
Is
there
any
feedback mechanism
that
can
prevent
the
system from extreme
fluctuations
in
PCO?
The
possibility
of a
feedback mechanism
seems
to lie in the
weathering portion
of the
cycle. Weathering
is
affected
by
temper-
ature,
rain, plants,
and
topography.
For
instance,
a
higher
Pco
2
results
in a
warmer
climate
with
more vegetation, which increases
the
rate
of
weathering
and
removes
COi
from
the
atmosphere, resulting
in a
negative feedback. Enhanced
magmatism
(source
of
CO?)
could lead
to
mountain
building;
the
resulting
steeper topography increases
the
rate
of
physical weathering and, consequently,
the
rate
of
chemical weathering
(sink
of
CC>2).
There
are
major uncertainties
in our
present understanding
of the
con-
trols
and
feedbacks
in the
regulation
of
atmospheric
CC>2.
Some
biological feedbacks
may be
attributed
to
Gaian
homeorrhesis,
but
proof
of
this
appears
to be
difficult.
9.4.4
CO:
and
Climate:
Pleistocene
and
Pliocene
Perhaps
the
most dramatic development
in the
climate
of the
Plio-Pleistocene
was the
occurrence
of ice
ages
during which Earth
was
colder
by
several degrees,
ice
sheets
covered much
of
North America
and
northern Europe,
and the
mean
sea
level
was
lower
by 120 m.
Figure
9.9
presents
the
global mean temperature
of
Earth since
the
Pleistocene
on
three time
scales:
(a) the
last million years,
(b) the
last
10,000
yr, and
(c) the
last 1000
yr. The
temperature variations have been estimated using isotopic
data from
deep
sea
cores
(the
CLIMAP
project)
and
isotopic measurements
of
water
ice in ice
cores
taken from polar regions. Variations
in the
time
series
in figure
9.9a
are
believed
to be
caused
by
astronomical forcing.
The
theory, developed
by
Milankovitch
early
in the
twentieth century, states that
the ice
ages
were
caused
by
small changes
in
insolation
at
high latitudes
due to
secular variations
of the
position
of the
Earth
in
its
orbit around
the sun at
vernal equinox,
the
tilt
of
Earth's rotational axis,
and
the
ellipticity
of
Earth's orbit.
These
variations have dominant
periods
of
about
20,
40, and 100 kyr and are
recorded
in the
observed data.
The
amplitudes
of the
forcing
function
are, however,
different
from those
of the
temperature variations. This
may be
explained
by the
nonlinear dynamics
of ice
growth
and
collapse.
The
changes
in
insolation
due to
astronomical forcing
are
very small.
It is now
known
that
the
Milankovitch effects
are
greatly enhanced
by
greenhouse
gases
such
as
CC>2
and
CH
4
.
Figure 9.10 shows
the
correlation between temperature,
CO:,
and
CH4
during
the
deglaciation
of the
penultimate glacial maximum (PGM) about
140
kyr
ago.
The
results suggest that during
the PGM the
atmospheric
CO2 and CH4
were
only
two-thirds
and
one-half
of the
interglacial values, respectively.
It is not
clear
from
the
generally
good
correlation
in
figure
9.10 that
the
greenhouse
gases
drove
climatic
changes.
The
only
piece
of
circumstantial evidence
in
favor
of
this possibility
is
in the
<5
18
O
values
of
atmospheric
O:
shown
in figure
9.10.
Without going into
the
details,
we
interpret
the
<5
I8
O
(of
©2)
data
to
reflect change
in ice
volume.
The PGM
results
suggest that
the ice
sheets
melt
after
the
rise
of
CO?,
CI-Lt,
and
temperature.
This tentative conclusion
has not
been confirmed
by
independent observations
or for
other glaciation periods.
We
should point
out
that even
if it is
proven that
CO:
and
other greenhouse
gases
drive climatic changes, questions
will
remain concerning what
drives
the
changes
in the
gases
and how the
gases
respond
to
changes
in the
orbital
parameters
of the
planet.