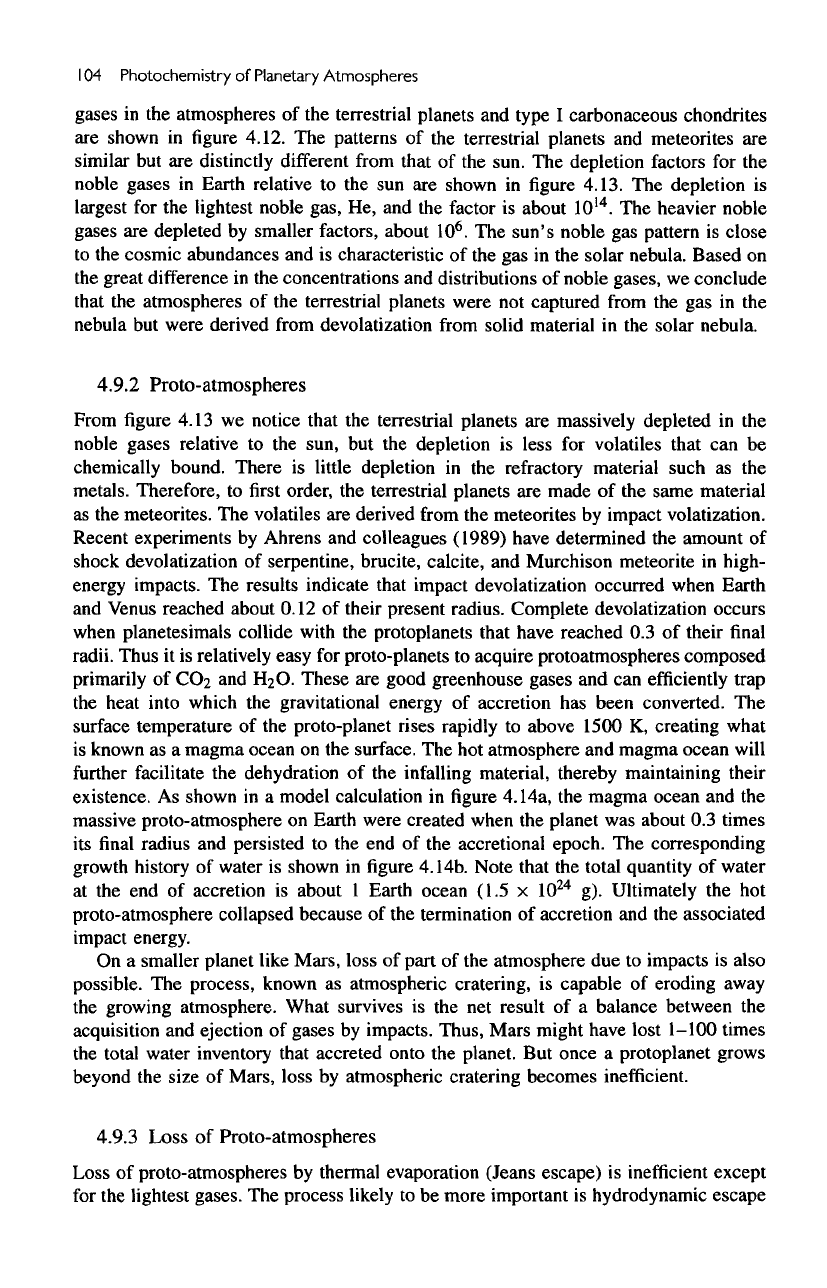
104
Photochemistry
of
Planetary
Atmospheres
gases
in the
atmospheres
of the
terrestrial planets
and
type
I
carbonaceous
chondrites
are
shown
in figure
4.12.
The
patterns
of the
terrestrial planets
and
meteorites
are
similar
but are
distinctly
different
from
that
of the
sun.
The
depletion factors
for the
noble
gases
in
Earth relative
to the sun are
shown
in figure
4.13.
The
depletion
is
largest
for the
lightest noble gas,
He, and the
factor
is
about
10
14
.
The
heavier noble
gases
are
depleted
by
smaller factors, about
10
6
.
The
sun's noble
gas
pattern
is
close
to
the
cosmic abundances
and is
characteristic
of the gas in the
solar nebula. Based
on
the
great
difference
in the
concentrations
and
distributions
of
noble gases,
we
conclude
that
the
atmospheres
of the
terrestrial planets were
not
captured
from
the gas in the
nebula
but
were derived
from
devolatization
from
solid material
in the
solar nebula.
4.9.2
Proto-atmospheres
From
figure
4.13
we
notice that
the
terrestrial planets
are
massively depleted
in the
noble
gases
relative
to the
sun,
but the
depletion
is
less
for
volatiles that
can be
chemically
bound. There
is
little depletion
in the
refractory material such
as the
metals. Therefore,
to first
order,
the
terrestrial planets
are
made
of the
same material
as
the
meteorites.
The
volatiles
are
derived
from
the
meteorites
by
impact volatization.
Recent experiments
by
Ahrens
and
colleagues
(1989)
have determined
the
amount
of
shock
devolatization
of
serpentine, brucite, calcite,
and
Murchison meteorite
in
high-
energy
impacts.
The
results indicate that impact devolatization occurred when Earth
and
Venus reached about
0.12
of
their present radius. Complete devolatization occurs
when
planetesimals collide with
the
protoplanets that have reached
0.3 of
their
final
radii. Thus
it is
relatively easy
for
proto-planets
to
acquire
protoatmospheres
composed
primarily
of CO2 and
H2O.
These
are
good greenhouse gases
and can
efficiently
trap
the
heat into which
the
gravitational energy
of
accretion
has
been converted.
The
surface
temperature
of the
proto-planet
rises rapidly
to
above 1500
K,
creating what
is
known
as a
magma ocean
on the
surface.
The hot
atmosphere
and
magma ocean will
further
facilitate
the
dehydration
of the
infalling
material, thereby maintaining their
existence.
As
shown
in a
model calculation
in figure
4.14a,
the
magma ocean
and the
massive
proto-atmosphere
on
Earth were created when
the
planet
was
about
0.3
times
its final
radius
and
persisted
to the end of the
accretional epoch.
The
corresponding
growth
history
of
water
is
shown
in figure
4.14b.
Note that
the
total
quantity
of
water
at
the end of
accretion
is
about
1
Earth ocean (1.5
x
10
24
g).
Ultimately
the hot
proto-atmosphere collapsed because
of the
termination
of
accretion
and the
associated
impact
energy.
On a
smaller planet like Mars, loss
of
part
of the
atmosphere
due to
impacts
is
also
possible.
The
process, known
as
atmospheric cratering,
is
capable
of
eroding away
the
growing atmosphere. What survives
is the net
result
of a
balance between
the
acquisition
and
ejection
of
gases
by
impacts. Thus, Mars might have lost 1-100 times
the
total water inventory
that
accreted onto
the
planet.
But
once
a
protoplanet grows
beyond
the
size
of
Mars, loss
by
atmospheric cratering becomes
inefficient.
4.9.3
Loss
of
Proto-atmospheres
Loss
of
proto-atmospheres
by
thermal evaporation (Jeans escape)
is
inefficient
except
for
the
lightest
gases.
The
process
likely
to be
more important
is
hydrodynamic
escape