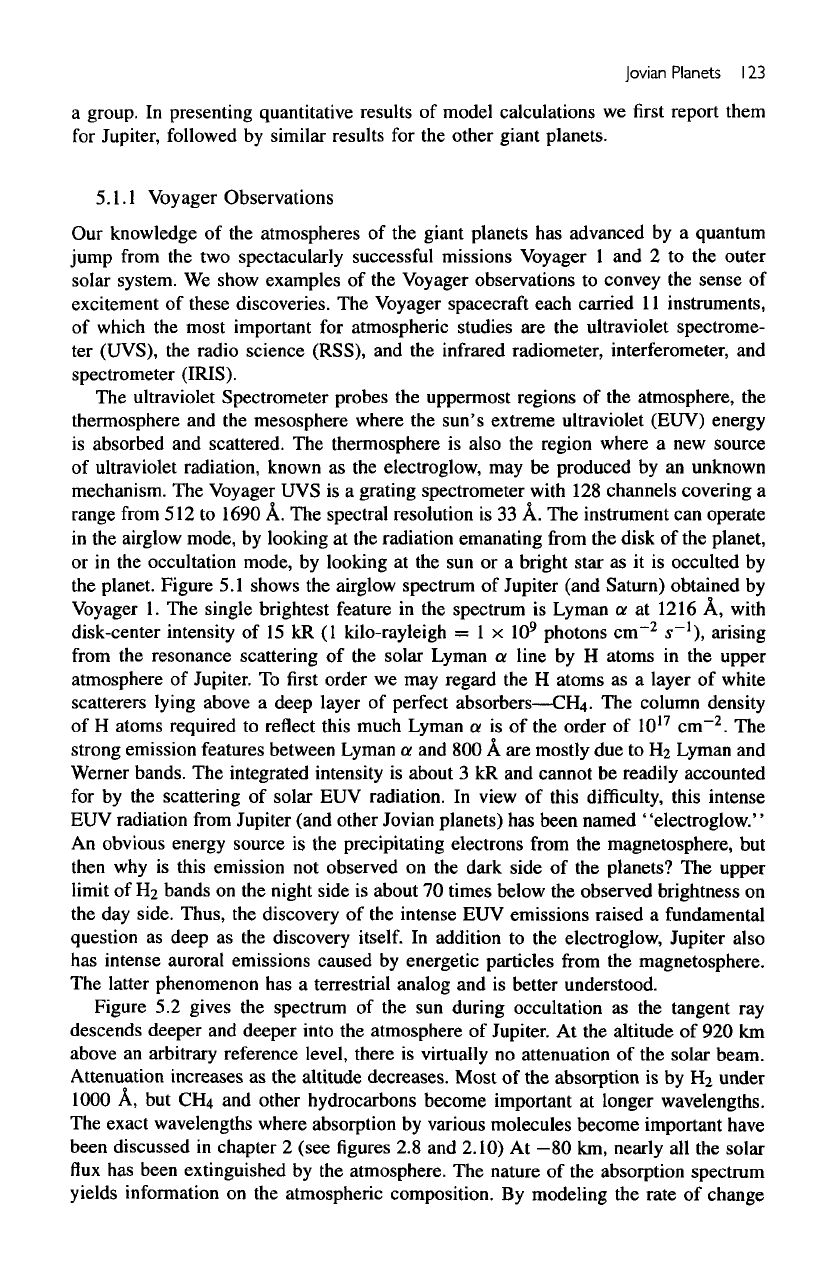
Jovian
Planets
123
a
group.
In
presenting quantitative results
of
model calculations
we first
report them
for
Jupiter, followed
by
similar results
for the
other giant planets.
5.1.1 Voyager Observations
Our
knowledge
of the
atmospheres
of the
giant planets
has
advanced
by a
quantum
jump
from
the two
spectacularly successful missions Voyager
1 and 2 to the
outer
solar system.
We
show examples
of the
Voyager observations
to
convey
the
sense
of
excitement
of
these discoveries.
The
Voyager spacecraft each
carried
11
instruments,
of
which
the
most important
for
atmospheric studies
are the
ultraviolet spectrome-
ter
(UVS),
the
radio science (RSS),
and the
infrared radiometer, interferometer,
and
spectrometer (IRIS).
The
ultraviolet Spectrometer probes
the
uppermost regions
of the
atmosphere,
the
thermosphere
and the
mesosphere where
the
sun's extreme ultraviolet
(EUV)
energy
is
absorbed
and
scattered.
The
thermosphere
is
also
the
region where
a new
source
of
ultraviolet radiation,
known
as the
electroglow,
may be
produced
by an
unknown
mechanism.
The
Voyager
UVS is a
grating spectrometer
with
128
channels covering
a
range
from
512
to
1690
A. The
spectral resolution
is 33 A. The
instrument
can
operate
in
the
airglow mode,
by
looking
at the
radiation emanating
from
the
disk
of the
planet,
or in the
occultation mode,
by
looking
at the sun or a
bright star
as it is
occulted
by
the
planet. Figure
5.1
shows
the
airglow spectrum
of
Jupiter (and Saturn) obtained
by
Voyager
1. The
single brightest feature
in the
spectrum
is
Lyman
a at
1216
A,
with
disk-center
intensity
of 15
kR
(1
kilo-rayleigh
= 1 x
10
9
photons
cm~
2
s~
]
),
arising
from
the
resonance scattering
of the
solar Lyman
a
line
by H
atoms
in the
upper
atmosphere
of
Jupiter.
To first
order
we may
regard
the H
atoms
as a
layer
of
white
scatterers
lying
above
a
deep
layer
of
perfect
absorbers—CH4.
The
column density
of
H
atoms required
to
reflect
this much Lyman
a is of the
order
of
10
17
cm~
2
.
The
strong emission features between Lyman
a and 800 A are
mostly
due to
Ha
Lyman
and
Werner bands.
The
integrated intensity
is
about
3 kR and
cannot
be
readily accounted
for
by the
scattering
of
solar
EUV
radiation.
In
view
of
this
difficulty,
this intense
EUV
radiation
from
Jupiter (and other Jovian planets)
has
been named
"electroglow."
An
obvious energy
source
is the
precipitating
electrons from
the
magnetosphere,
but
then
why is
this emission
not
observed
on the
dark side
of the
planets?
The
upper
limit
of H2
bands
on the
night
side
is
about
70
times below
the
observed brightness
on
the
day
side. Thus,
the
discovery
of the
intense
EUV
emissions raised
a
fundamental
question
as
deep
as the
discovery itself.
In
addition
to the
electroglow, Jupiter also
has
intense auroral emissions caused
by
energetic
particles
from
the
magnetosphere.
The
latter phenomenon
has a
terrestrial analog
and is
better understood.
Figure
5.2
gives
the
spectrum
of the sun
during occultation
as the
tangent
ray
descends
deeper
and
deeper
into
the
atmosphere
of
Jupiter.
At the
altitude
of 920 km
above
an
arbitrary reference level, there
is
virtually
no
attenuation
of the
solar
beam.
Attenuation
increases
as the
altitude
decreases.
Most
of the
absorption
is by
H
2
under
1000
A, but CH4 and
other hydrocarbons
become
important
at
longer wavelengths.
The
exact wavelengths where absorption
by
various molecules
become
important have
been discussed
in
chapter
2
(see
figures 2.8 and
2.10)
At
—80
km,
nearly
all the
solar
flux has
been extinguished
by the
atmosphere.
The
nature
of the
absorption spectrum
yields
information
on the
atmospheric composition.
By
modeling
the
rate
of
change