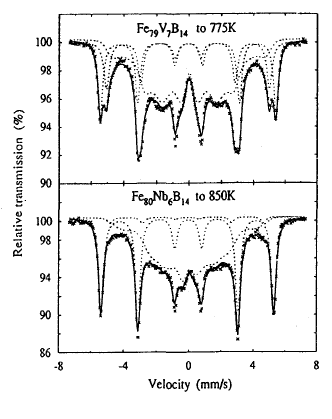
To illustrate the quantitative fitting of spectra comprising both broad
amorphous and sharper nano-crystalline components, we show in Figure 5.28
the room temperature Mössbauer spectra of (i) Fe
79
V
7
B
14
and (ii) Fe
80
Nb
6
B
14
ribbons, prepared fully amorphous by the melt-spinning technique and
subsequently partially crystallized to produce bcc-Fe(V,Nb,B) nanocrysta-
llites, embedded in an amorphous matrix [49]. These spectra show the
combined features of the broad amorphous phase and the sharper nano-
crystalline precipitates. Fitting these combinations allow one to deduce the
volume fractions of the amorphous and nano-crystalline phases in these
materials. Such data are important in the development of a comprehensive
model for the random anisotropy in such commercially important soft-
magnetic materials [16, 50].
Figure 5.28
57
Fe spectra of partially crystallized (top) Fe
79
V
7
B
14
and (bottom) Fe
80
Nb
6
B
14
ribbons [49].
Mössbauer spectroscopy also provides a simple method for estimating the
magnetic ordering temperature of a material. The “thermal scan” technique
consists of switching off the velocity drive unit and counting for fixed periods
of time in the zero-velocity channel at various temperatures. As the
temperature increases, the magnetic spectrum collapses towards a quadrupole
doublet or a singlet, depending on the crystallographic site symmetry. Thus,
the J absorption increases at zero-velocity and the number of counts registered
in the given time period falls. This is illustrated in Figure 5.29 where we show
spectra of amorphous Fe
90
Sc
10
obtained at 4.2 K and 300 K [51]. The
temperature dependence of the average hyperfine field yields a Curie
temperature of 98 K. The spectrum at 300 K is a paramagnetic, broad doublet
due to the distribution of the electric quadrupole splitting. This figure also
5.3 Applications 243
shows the Curie temperature determination using the thermal scan technique,