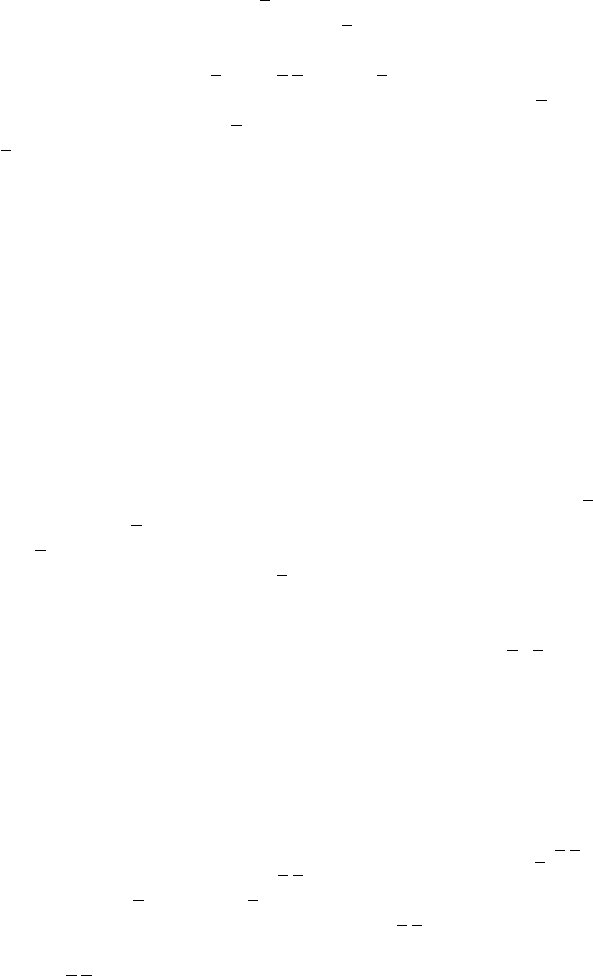
Titanium alloys: modelling of microstructure288
processes of decohesion (along
(1210)
plane in our case). Here, the trace of
a macrocrack plane coincides with the
[1321]
line direction. In a Ti
3
Al
single crystal with operative basal slip, cracks propagate along macroscopic
habit planes parallel to
{1012}, {21 13} and {4311}
. Taking into account
only these types of planes, it is possible to demonstrate that the
[1321]
line
direction coincides with the
(2113)
plane projection into the surface foil
(1011)
. Thus, the accumulation of shear microcracks in (0001) slip bands,
being the initiating mechanism of macrocleavage, ultimately determines the
low plasticity of a single crystal Ti
3
Al with operative basal slip.
It is noted that stresses generated by superdislocations in a slip band
might promote both microcrack nucleation and transversal slip of screw
superdislocations in the prism plane. Formation of plastic zones in the prism
plane leads to microcrack blunting and coming to a stop. These processes are
observed experimentally (Fig. 11.6). Therefore, in the slip band within several
parallel basal planes, a microcrack system forms. A main crack forms as a
result of the coalescence of these microcracks.
The type of the forming macrocleavage surface is determined by the
values of applied stresses and decohesion energy for the crack plane. The
experimental data prove that decohesion occurs in pyramidal planes. Normals
to the experimentally observed pyramidal planes lie on the stereographical
projection near the arc of the big circle which depicts the trace of the
(1011)
plane with the
[1012]
normal. When a single crystal is compressed along
the
[1012]
direction, the maximum normal tensile stress appears in the
planes whose normals lie in the
(1011)
plane, i.e. they belong to the above
arc of the big circle. Thus, a cleavage in the pyramidal planes with maximum
normal stress is observed experimentally. Although external normal stress is
high, we did not observe microcracks in prism planes of the
{1210}
type,
for instance, suggesting that they are caused by the difference in cohesion
characteristic of these planes (cleavage stress, surface energy, etc.). This
matter requires additional investigation.
There is one more significant element which facilitates microcrack
nucleation. In this section, we have described the model of shear microcrack
nucleation in the basal plane. According to this model, a microcrack nucleates
as a result of coalescence of several a-superdislocations of screw orientation,
i.e. the top of a formed microcrack is parallel to the Burgers vector
1
3
1120〈〉
of superdislocation. This direction <
1120
> belongs to cleavage microfacets
on the (0001),
{1012}
and
{1011}
planes of the zigzag cracks, observed
experimentally (Fig. 11.4 and Table 11.2). The
{1 123}
plane is often the
main plane of a crack. One can suppose that in the top of the crack in the
planes of
{1 123}
type, a plastic zone does not form by the dislocations of
prism slip systems. This prevents the blunting of the crack and promotes its
spreading through the bulk of the crystal.