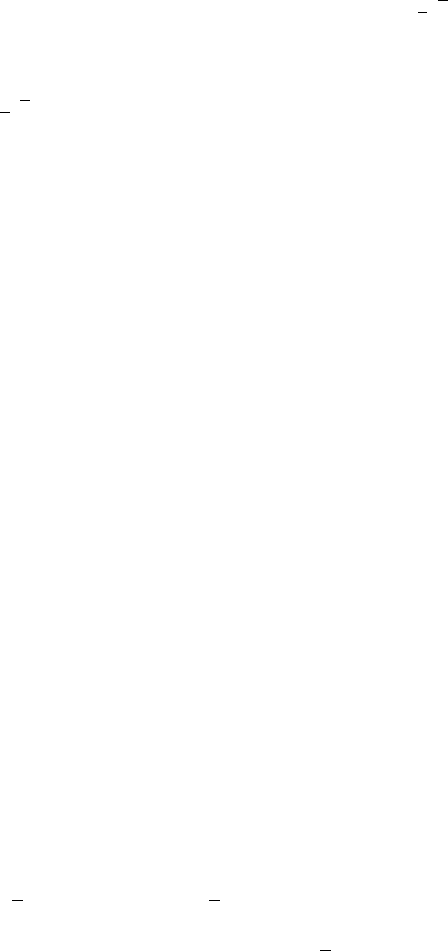
Crystallographic and fracture behaviour of titanium aluminide 287
computer modelling of the core structure of the superdislocations
1
3
2110〈〉
and <110> in these two superstructures. A superdislocation with the Burgers
vector <110> splits in the octahedral plane into four Shockley partial
dislocations, connected by APB bands and CSF, similarly to the
superdislocations
1
3
2110〈〉
in the basal plane. The core structure of Shockley
partial dislocations differs essentially in these cases. For a screw
superdislocation in Ti
3
Al, the core of a Shockley partial dislocation is nonplanar,
and noticeable displacements take place in the prism plane (Fig. 11.9), whereas
for L1
2
superstructure the displacements near the core of Shockley superpartial
dislocations are localised in the octahedron plane. This makes the configuration
considered to be a gliding one for L1
2
superstructure, while the motion of a
superpartial dislocation is impeded for DO
19
superstructure. The stress field
of other a-superdislocations in a slip band of the basal plane causes the
lowering of Peierls stress, and, apparently, results in coarse slip band formation
in this plane.
External stress and the stress imposed on a dislocation in the slip band
might change the core structure of the dislocation. For instance, in L1
2
superstructure, the stress imposed by the array dislocations might promote
the Shockley partial dislocations recombination, as a result of a decreasing
of the distance between the partial dislocations, and facilitate the process of
cross-slip of screw superdislocations. For Ti
3
Al, this stress can both promote
and impede the process recombination and cross-slip of a superpartial
dislocation, depending on the relation between the components of stress
effective in the basal and prism planes. If stress, caused by the other dislocations
in the slip band, promotes the widening of Shockley partial dislocation splitting
in the prism plane, the processes of recombination and cross-slip become
difficult, but coalescence of a-superdislocations with microcracks nucleation
by the mechanism introduced here is easy to initiate. If the stress in the prism
plane is small, it is possible to activate thermally the process of a-
superdislocations cross-slip. Thus, in the slip band both processes of shear
microcracks nucleation and slip-cross process might take place. In fact, the
cross-slip of the a-superdislocations into the prism plane in a slip band when
the basal slip is operative has been observed experimentally, along with
shear microcrack nucleation.
In Section 11.2, the surface of macrocleavage is shown to be stepped. One
of the steps is parallel to the basal plane; the trace of the other one on the
crystal surface
(1011)
coincides with
[1210]
line direction (Fig. 11.3b).
Thus, the cleavage-like crack propagation might be due to consecutive processes
of shear along the basal plane and decohesion in the
(1210)
prism plane. It
might be suggested that the microcracks of shear type nucleate first in the
basal planes. These microcracks nucleate in the places of high concentration
of local stress in one or several parallel slip bands. When the density of shear
microcracks is comparatively high, the macrocrack is nucleated due to some