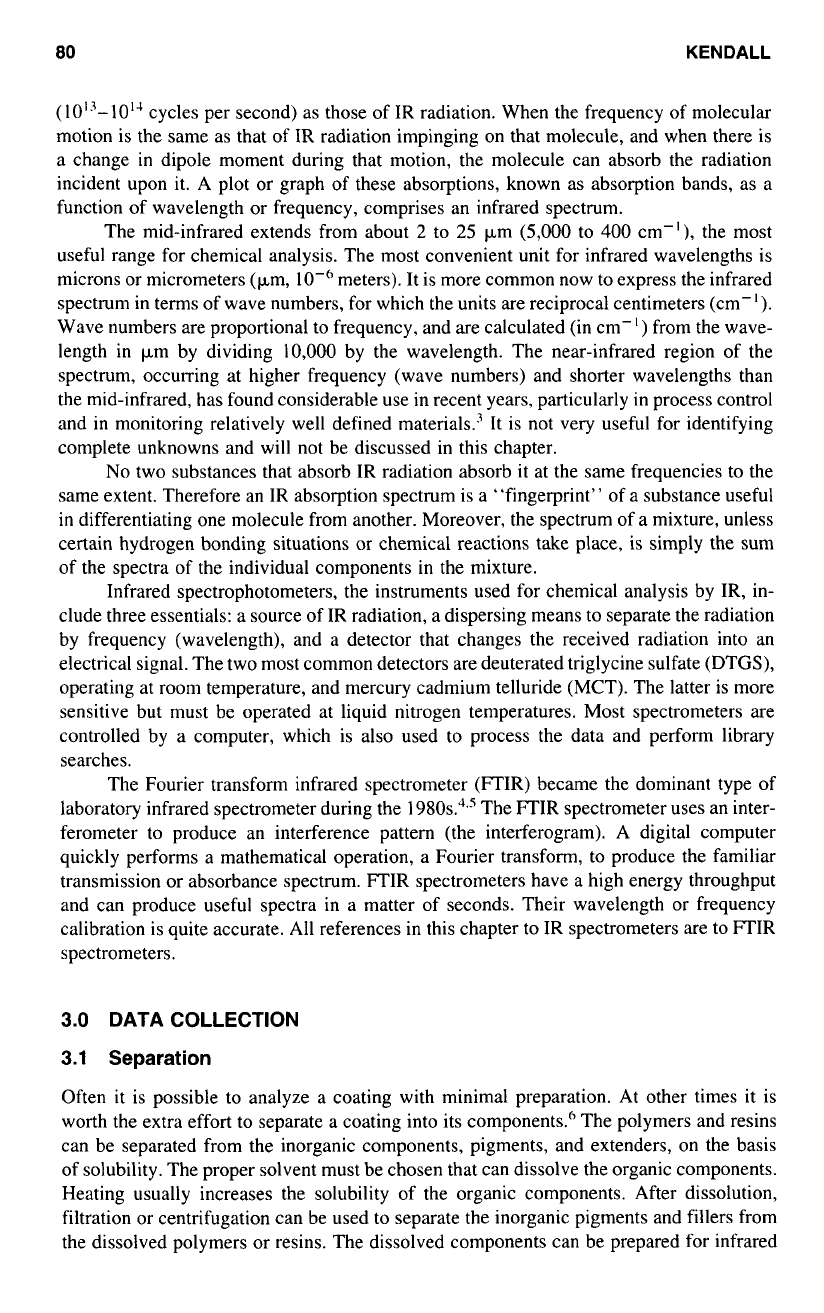
80
KENDALL
(
10'3-10'' cycles per second) as those of
IR
radiation. When the frequency
of
molecular
motion is the same as that
of
IR
radiation impinging on that molecule, and when there is
a change
in
dipole moment during that motion, the molecule can absorb the radiation
incident upon it. A plot or graph
of
these absorptions, known as absorption bands, as a
function of wavelength or frequency, comprises an infrared spectrum.
The mid-infrared extends from about
2
to
25
pm
(5,000
to
400
cm-'), the most
useful range for chemical analysis. The most convenient unit for infrared wavelengths is
microns or micrometers (pm,
lo-('
meters). It is more common now
to
express the infrared
spectrum in terms of wave numbers, for which the units are reciprocal centimeters (cm-').
Wave numbers are proportional to frequency, and are calculated (in cm-') from the wave-
length
in
pm by dividing 10,000 by the wavelength. The near-infrared region of the
spectrum, occurring at higher frequency (wave numbers) and shorter wavelengths than
the mid-infrared, has found considerable use in recent years, particularly in process control
and in monitoring relatively well defined materials.' It is not very useful for identifying
complete unknowns and will not be discussed in this chapter.
No
two substances that absorb
IR
radiation absorb it at the same frequencies to the
same extent. Therefore an
IR
absorption spectrum is a "fingerprint" of a substance useful
in differentiating one molecule from another. Moreover, the spectrum of a mixture, unless
certain hydrogen bonding situations or chemical reactions take place, is simply the sum
of the spectra of the individual components in the mixture.
Infrared spectrophotometers, the instruments used for chemical analysis by
IR,
in-
clude three essentials: a source
of
IR
radiation, a dispersing means to separate the radiation
by frequency (wavelength), and a detector that changes the received radiation into an
electrical signal. The two most common detectors are deuterated triglycine sulfate (DTGS),
operating at room temperature, and mercury cadmium telluride (MCT). The latter is more
sensitive but must be operated at liquid nitrogen temperatures. Most spectrometers are
controlled by a computer, which is also used to process the data and perform library
searches.
The Fourier transform infrared spectrometer
(FTIR)
became the dominant type
of
laboratory infrared spectrometer during the
1980s."'
The
FTIR
spectrometer uses an inter-
ferometer to produce an interference pattern (the interferogram). A digital computer
quickly performs a mathematical operation, a Fourier transform, to produce the familiar
transmission or absorbance spectrum.
FTIR
spectrometers have a high energy throughput
and can produce useful spectra in a matter of seconds. Their wavelength or frequency
calibration is quite accurate. All references in this chapter to
IR
spectrometers are to
FTIR
spectrometers.
3.0
DATA COLLECTION
3.1
Separation
Often it is possible to analyze a coating with minimal preparation. At other times it is
worth the extra effort to separate a coating into its components." The polymers and resins
can be separated from the inorganic components, pigments, and extenders,
on
the basis
of
solubility. The proper solvent must be chosen that can dissolve the organic components.
Heating usually increases the solubility of the organic components. After dissolution,
filtration or centrifugation can be used to separate the inorganic pigments and fillers from
the dissolved polymers or resins. The dissolved components can be prepared for infrared