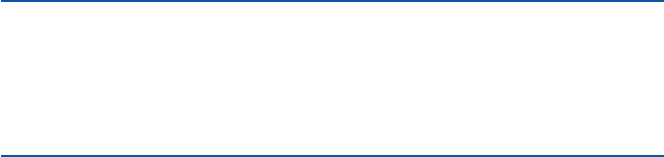
266
21 Are Atoms and Molecules for Real? Can We See Them?
very good pictures of molecules with atomic level resolution in some cases, and
really convince us that, yes, atoms and molecules do exist, and moreover, that they
do look like how chemists have been saying they do. What more do you need to be
convinced?
21.3 X-Ray Diffraction: Atomic Structure of Large Molecular
Compounds and Ionic Compounds
The scanning probe microscopes are still unable to resolve atomic structures of such
complicated large molecules as proteins and DNAs. The atomic structures of these
large molecules (as well as smaller molecules and ionic compounds) are now almost
routinely determined by X-ray crystallography. This technique is much older than
SPM. However, the way this technique produces the atomic structure of a molecule is
not as straightforward as electron microscopy or scanning probe microscopy. Yet, the
structures obtained by this techniques, particularly those of smaller molecules and ionic
compounds, have well been verified by many other methods and experience. Therefore,
the atomic structure of a large molecule obtained by this method is also now well
believed to represent the correct structure of the compound. It is true, though, that the
structure is valid only for the solid crystal state to which this method is applied.
We will explain very briefly how it is done with a few examples. X-ray is a light
of very short wavelength. For example, when an electron beam is directed to a copper
metal, the copper metal produces an X-ray of 154 pm (1.54 Å). This is the source of
light (X-ray, that is). The light (any light) shone on a compound will be scattered by
the electron clouds (of atoms) of the compound. When the atoms are arranged regu-
larly as in a crystal, this scattered light will show a pattern. This is called diffraction
pattern, and is related to the crystal structure. The diffraction pattern can be photo-
graphed or now digitally recorded. The pattern consists of spots (where the diffracted
X-ray hits) of differing intensities. The intensity of a spot is related to the electron
cloud density that scatters X-ray. (The more dense electron cloud scatters X-ray more
strongly; thus the spot will be more intense). Therefore, we can reconstruct how the
electron clouds are distributed throughout space (in a crystal) by analyzing the dif-
fraction pattern including intensities. The result can be expressed as an electron den-
sity map (in three dimensions), and that can then be reinterpreted as an aggregate of
atoms. Softwares have now been developed to convert the diffraction pattern to the
atomic arrangements in a molecule. The resolution obtained is now routinely about
1.5–2 Å (150–200 pm = 0.15–0.2 nm). Hence, we can distinguish atoms in molecules,
as the inter-atomic distances in molecules are around 1–2 Å (0.1–0.2 nm).
The actual process is now fairly automated, but one of the basic problems is to
obtain a single crystal of an appropriate size. Many biologically interesting samples
such as proteins and DNAs are difficult to crystallize, and researchers would spend
days, months, and even years to get single crystals suitable for X-ray crystallographic
studies. Many other problems prevent X-ray studies of crystal structures from becom-
ing a truly routine technique. But it is not appropriate to pursue this issue further here.
Below we display a few protein structures (Figs. 21.10–21.12) determined by
X-ray crystallography. Figure 21.10 shows three different modes of expressing