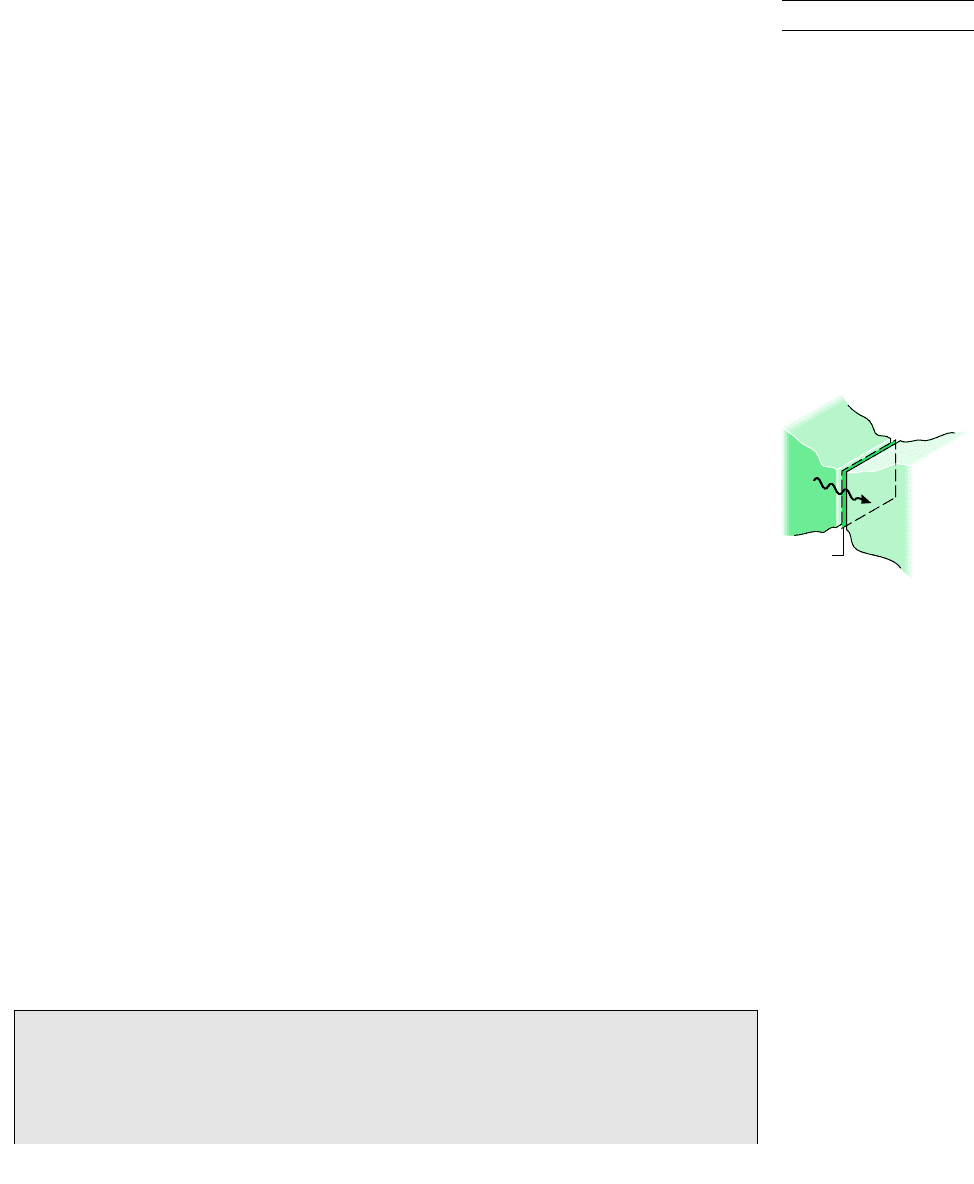
5.2 Identifying Irreversibilities 181
As a system undergoes a process, irreversibilities may be found within the system as
well as within its surroundings, although in certain instances they may be located pre-
dominately in one place or the other. For many analyses it is convenient to divide the ir-
reversibilities present into two classes. Internal irreversibilities are those that occur within
the system. External irreversibilities are those that occur within the surroundings, often
the immediate surroundings. As this distinction depends solely on the location of the
boundary, there is some arbitrariness in the classification, for by extending the boundary
to take in a portion of the surroundings, all irreversibilities become “internal.” Nonethe-
less, as shown by subsequent developments, this distinction between irreversibilities is
often useful.
Engineers should be able to recognize irreversibilities, evaluate their influence, and develop
practical means for reducing them. However, certain systems, such as brakes, rely on the
effect of friction or other irreversibilities in their operation. The need to achieve profitable
rates of production, high heat transfer rates, rapid accelerations, and so on invariably dictates
the presence of significant irreversibilities. Furthermore, irreversibilities are tolerated to some
degree in every type of system because the changes in design and operation required to reduce
them would be too costly. Accordingly, although improved thermodynamic performance can
accompany the reduction of irreversibilities, steps taken in this direction are constrained by
a number of practical factors often related to costs.
for example. . . consider two bodies at different temperatures that are able to
communicate thermally. With a finite temperature difference between them, a spontaneous
heat transfer would take place and, as discussed previously, this would be a source of
irreversibility. It might be expected that the importance of this irreversibility would di-
minish as the temperature difference approaches zero, and this is the case. From the study
of heat transfer (Sec. 2.4), we know that the transfer of a finite amount of energy by heat
between bodies whose temperatures differ only slightly would require a considerable
amount of time, a larger (more costly) heat transfer surface area, or both. To eliminate this
source of irreversibility, therefore, would require an infinite amount of time and/or an
infinite surface area.
Whenever any irreversibility is present during a process, the process must necessarily be ir-
reversible. However, the irreversibility of the process can be demonstrated using the
Kelvin–Planck statement of the second law and the following procedure: (1) Assume there
is a way to return the system and surroundings to their respective initial states. (2) Show that
as a consequence of this assumption, it would be possible to devise a cycle that produces
work while no effect occurs other than a heat transfer from a single reservoir. Since the ex-
istence of such a cycle is denied by the Kelvin–Planck statement, the initial assumption must
be in error and it follows that the process is irreversible. This approach can be used to demon-
strate that processes involving friction (see box), heat transfer through a finite temperature
difference, the unrestrained expansion of a gas or liquid to a lower pressure, and other effects
from the list given previously are irreversible. However, in most instances the use of the
Kelvin–Planck statement to demonstrate the irreversibility of processes is cumbersome. It is
normally easier to use the entropy production concept (Sec. 6.5).
internal and external
irreversibilities
DEMONSTRATING IRREVERSIBILITY: FRICTION
Let us use the Kelvin–Planck statement to demonstrate the irreversibility of a process
involving friction. Consider a system consisting of a block of mass m and an inclined
plane. Initially the block is at rest at the top of the incline. The block then slides down