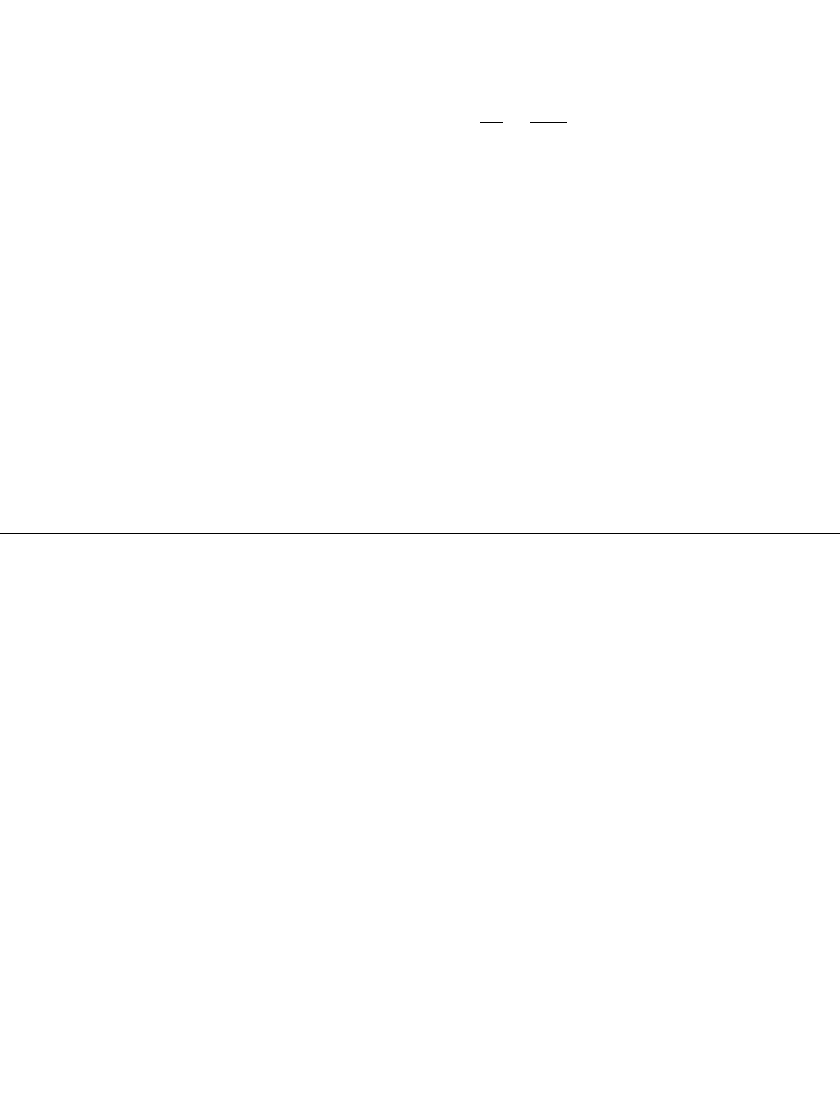
ELECTROCHEMICAL PRINCIPLES AND REACTIONS 2.5
When conditions are other than in the standard state, the voltage E of a cell is given by the
Nernst equation,
cd
RT a a
CD
0
E ⫽ E ⫺ ln (2.6)
ab
nF a a
AB
where a
i
⫽ activity of relevant species
R
⫽ gas constant
T
⫽ absolute temperature
The change in the standard free energy
⌬G
0
of a cell reaction is the driving force which
enables a battery to deliver electrical energy to an external circuit. The measurement of the
electromotive force, incidentally, also makes available data on changes in free energy, entro-
pies and enthalpies together with activity coefficients, equilibrium constants, and solubility
products.
Direct measurement of single (absolute) electrode potentials is considered practically im-
possible.
1
To establish a scale of half-cell or standard potentials, a reference potential ‘‘zero’’
must be established against which single electrode potentials can be measured. By conven-
tion, the standard potential of the H
2
/H
⫹
(aq) reaction is taken as zero and all standard
potentials are referred to this potential. Table 2.2 and Appendix B list the standard potentials
of a number of anode and cathode materials.
2.3 ELECTRODE PROCESSES
Reactions at an electrode are characterized by both chemical and electrical changes and are
heterogeneous in type. Electrode reactions may be as simple as the reduction of a metal ion
and incorporation of the resultant atom onto or into the electrode structure. Despite the
apparent simplicity of the reaction, the mechanism of the overall process may be relatively
complex and often involves several steps. Electroactive species must be transported to the
electrode surface by migration or diffusion prior to the electron transfer step. Adsorption of
electroactive material may be involved both prior to and after the electron transfer step.
Chemical reactions may also be involved in the overall electrode reaction. As in any reaction,
the overall rate of the electrochemical process is determined by the rate of the slowest step
in the whole sequence of reactions.
The thermodynamic treatment of electrochemical processes presented in Sec. 2.2 de-
scribes the equilibrium condition of a system but does not present information on nonequi-
librium conditions such as current flow resulting from electrode polarization (overvoltage)
imposed to effect electrochemical reactions. Experimental determination of the current-
voltage characteristics of many electrochemical systems has shown that there is an exponen-
tial relation between current and applied voltage. The generalized expression describing this
relationship is called the Tafel equation,
⫽ a Ⳳ b log i (2.7)
where
⫽ overvoltage
i
⫽ current
a, b
⫽ constants
Typically, the constant b is referred to as the Tafel slope.
The Tafel relationship holds for a large number of electrochemical systems over a wide
range of overpotentials. At low values of overvoltage, however, the relationship breaks down
and results in curvature in plots of
versus log i. Figure 2.2 is a schematic presentation of
a Tafel plot, showing curvature at low values of overvoltage.