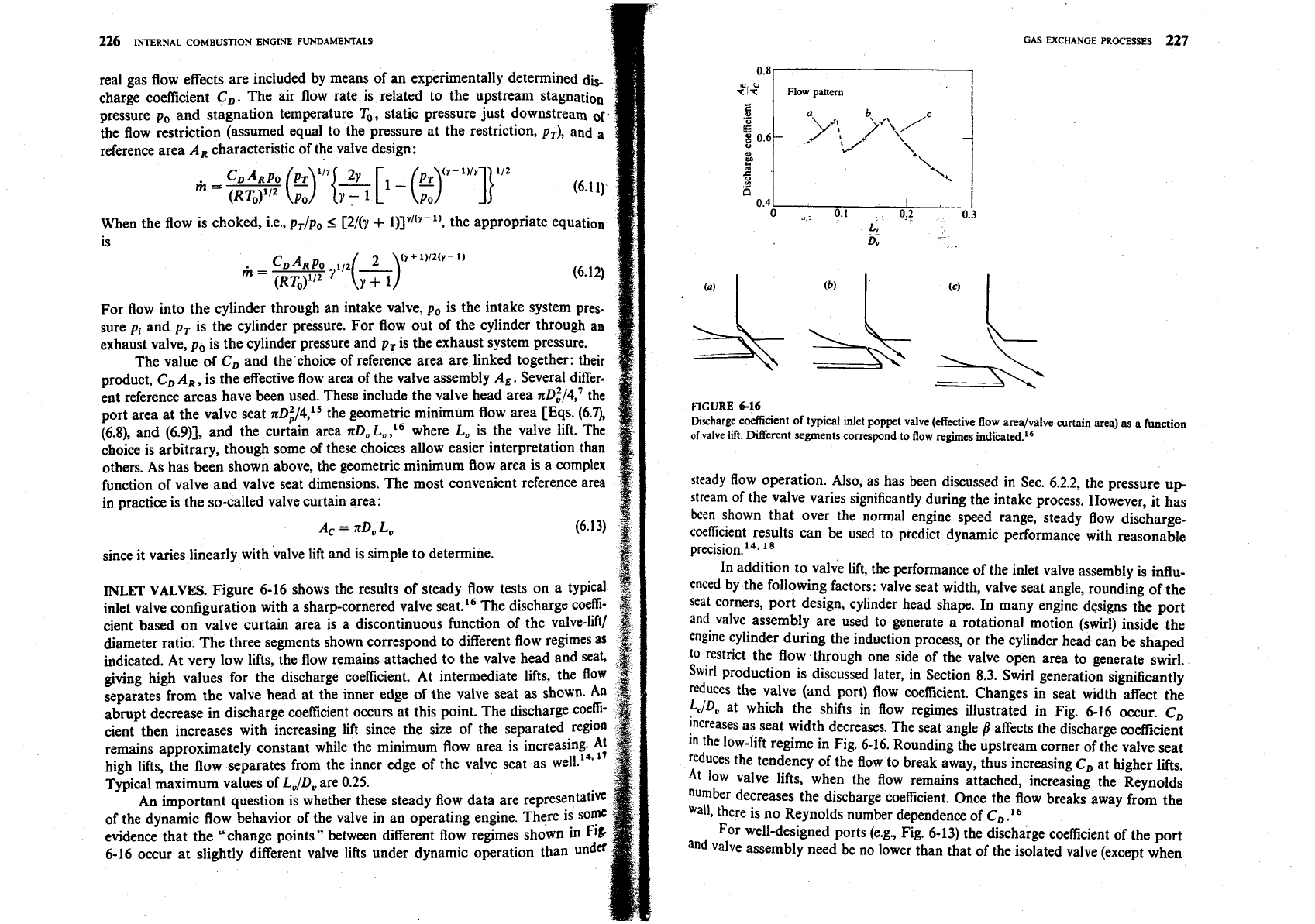
m=-
(6.1
1)
When the flow is choked, i.e., pT/po
5
[2/(y
+
l)]Y1'Y-l', the appropriate equation
is
(Y
+
1)/2(Y
-
1)
m=-
(6.12)
For flow into the cylinder through an intake valve, p, is the intake system pres-
sure
pi and p, is the cylinder pressure. For flow out of the cylinder through an
exhaust valve, p, is the cylinder pressure and
p,
is the exhaust system pressure.
The value of
C,
and the choice of reference area are linked together: their
product,
C,
A,,
is the effective flow area of the valve assembly
A,.
Several differ-
ent reference areas have been used. These include the valve head area nD;/4,' the
port area at the valve seat nD;/4,l5 the geometric minimum flow area [Eqs. (6.7),
(6.8), and (6.9)], and the curtain area RD,L,,'~ where L, is the valve lift. The
choice is arbitrary, though some of these choices allow easier interpretation than
others. As has been shown above, the geometric minimum flow area is a complex
function of valve and valve seat dimensions. The most convenient reference area
in practice is the so-called valve curtain area:
226
INTERNAL COMBUSTION ENGINE FUNDAMENTALS
real gas flow effects are included by means of an experimentally determined di,
charge coefficient
C,.
The air flow rate is related to the upstream stagnation
pressure
p,
and stagnation temperature
To,
static pressure just downstream
of.
the flow restriction (assumed equal to the pressure at the restriction, p,), and
a
reference area
A,
characteristic of the valve design:
since it varies linearly with valve lift and is simple to determine.
INLET VALVES.
Figure 6-16 shows the results of steady flow tests on a typical
inlet valve configuration with a sharp-cornered valve seat.16 The discharge coefli-
cient based on valve curtain area is a discontinuous function of the valve-lift/
diameter ratio. The three segments shown correspond to different flow regimes
as
indicated. At very low lifts, the flow remains attached to the valve head and seat,
giving high values for the discharge coefficient. At intermediate lifts, the flow
separates from the valve head at the inner edge of the valve seat as shown.
An
abrupt decrease in discharge coefficient occurs at this point. The discharge coefli-
cient then increases with increasing lift since the size of the separated region
remains approximately constant while the minimum flow area is increasing. At
high lifts, the flow rparates from the inner edge of the valve seat as
Typical maximum values of L JD, are 0.25.
An important question is whether these steady flow data are representative
of the dynamic flow behavior of the valve in an operating engine. There is some
evidence that the "change points" between different flow regimes shown in Fig
6-16 occur at slightly different valve lifts under dynamic operation than unda
FlCURE
6-16
Discharge coefficient of typical inlet poppet valve (effective flow area/valve curtain area) as a function
of
valve
lift.
Different segments correspond to flow regimes indicated.16
steady flow operation. Also, as has been discussed in Sec. 6.2.2, the pressure up-
stream of the valve varies significantly during the intake process. However, it has
hen shown that over the normal engine speed range, steady flow discharge-
coefficient results can
be
used to predict dynamic performance with reasonable
preci~ion.'~.
In addition to valve lift, the performance of the inlet valve assembly
is
influ-
enced by the following factors: valve seat width, valve seat angle, rounding of the
scat corners, port design, cylinder head shape. In many engine designs the port
and valve assembly are used to generate a rotational motion (swirl) inside the
engine cylinder during the induction process, or the cylinder head can be shaped
to restrict the flow through one side of the valve open area to generate swirl.
Swirl production is discussed later, in Section 8.3. Swirl generation significantly
reduces the valve (and port) flow coeficient. Changes in seat width affect the
LJD,
at which the shifts in flow regimes illustrated in Fig. 6-16 occur.
CD
increases as seat width decreases. The seat angle
B
affects the discharge coefficient
in
the low-lift regime in Fig. 6-16. Rounding the upstream corner of the valve seat
reduces the tendency of the flow to break away, thus increasing
CD
at higher lifts.
At
low valve lifts, when the flow remains attached, increasing the Reynolds
number decreases the discharge coefficient. Once the flow breaks away from the
Wall, there is no Reynolds number dependence of
CD.I6
For well-designed ports (e.g., Fig. 6-13) the discharge coefficient of the port
and valve assembly need
be
no lower than that of the isolated valve (except when