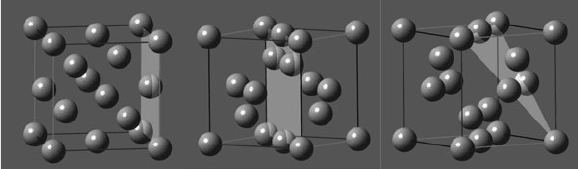
In addition, no native oxide forms on GaAs, which increases the complexity and cost
of production. Lastly, GaAs has a much lower thermal conductivity relative to Si,
resulting in lower packing densities at a time when Moore’s Law demands signifi-
cant increases. Germanium and other III–V such as InP, InAs, or InSb have also been
suggested to replace Si in future technology nodes due to their higher electron
mobilities (3,900, 5,400, 40,000, and 77,000 cm
2
V
1
s
1
, respectively) , of interest
for nMOS devi ce applications. Germanium also has a very high hole mobility
(1,900 cm
2
V
1
s
1
vs. 430 (Si), 400 (G aAs), 200 (InP), 500 (InAs), and 850
(InSb)), which could be useful for pMOS devices.
[18]
A substrate candidate that is used for high-speed optical networking and inexpen-
sive, lightweight personal communication devices is SiGe (“siggie”). Whereas Si
does not operate at frequencies above a few GHz, SiGe semiconductors have speeds
up to 120 GHz, which increases current speeds by up to a factor of four.
[19]
The
added processing cost will not result in the replacement of Si for CMOS applica-
tions; however, SiGe might replace III–V semiconductors (e.g., InP, GaAs) for niche
future applications such as watch-size cellular phones, collision-avoidance radar
systems, wireless ICs, and low-power radio-frequency (RF) chips.
Regardless of bulk Si, epi, or SOI subst rates, a key consideration that is para-
mount toward subsequent processing steps is the crystal orientation employed.
Three important crystal planes for silicon include Si(100), Si(110), and Si(111) –
Figure 4.35. The surface atomic densities increase in the order Si(100) < Si(110)
< Si(111). Empirically, this translates to available Si–Si bond densities of
6.77 10
14
, 9.59 10
14
, and 11.76 10
14
cm
2
, respectively.
[20]
Hence, the
rates required to remove (etch), or react with, surface atoms (e.g., thin-film deposi-
tion) should follow the reverse order as above. However, the Si(110) orientation
etches fastest due to its more corrugated structure, relative to the other atomically
flat surfaces (Figure 4.36). To illustrate this effect, exposure of a wafer to a 40%
alcoholic KOH solution results in Si etching at rates of 13,000, 6,000, and 90 A
˚
min
1
, for the Si(110), Si(100), and Si(1 11) planes, respectively.
Since bonds are broke n during Si wafer formation from the bulk ingot, atoms at
the surface will have a lower coordination number, resulting in a higher surface
energy and greater reactivity (Figure 4.37). One way to remove these surface defects
is through passivation. For example, a large number of dangling bonds are tied up
Figure 4.35. Unit cell representations of silicon showing the (100), (110), and (111) planes, respectively.
274 4 Semiconductors