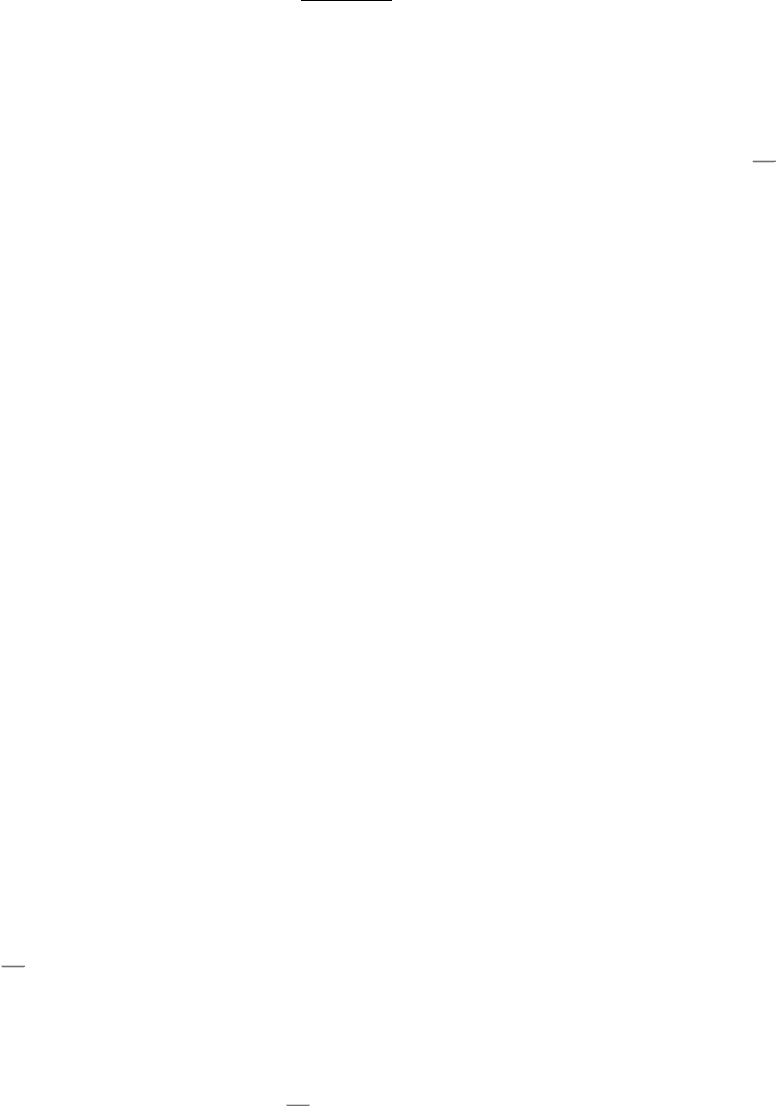
P. Kraulis. 1991. MOLSCRIPT: A program to produce both detailed and schematic plots of protein structures J. Appl.
Cryst. 24: 946-950.
T. Ferrin, C. Huang, L. Jarvis, and R. Langridge. 1988. The MIDAS display system J. Mol. Graphics 6: 13-27.
D.C. Richardson and J.S. Richardson. 1994. Kinemages: Simple macromolecular graphics for interactive teaching and
publication Trends Biochem. Sci. 19: 135-138. (PubMed)
I. The Molecular Design of Life
4. Exploring Proteins
In the preceding chapter, we saw that proteins play crucial roles in nearly all biological processes
in catalysis, signal
transmission, and structural support. This remarkable range of functions arises from the existence of thousands of
proteins, each folded into a distinctive three-dimensional structure that enables it to interact with one or more of a highly
diverse array of molecules. A major goal of biochemistry is to determine how amino acid sequences specify the
conformations of proteins. Other goals are to learn how individual proteins bind specific substrates and other molecules,
mediate catalysis, and transduce energy and information.
The purification of the protein of interest is the indispensable first step in a series of studies aimed at exploring protein
function. Proteins can be separated from one another on the basis of solubility, size, charge, and binding ability. When a
protein has been purified, the amino acid sequence can be determined. The strategy is to divide and conquer, to obtain
specific fragments that can be readily sequenced. Automated peptide sequencing and the application of recombinant
DNA methods are providing a wealth of amino acid sequence data that are opening new vistas. To understand the
physiological context of a protein, antibodies are choice probes for locating proteins in vivo and measuring their
quantities. Monoclonal antibodies able to probe for specific proteins can be obtained in large amounts. The synthesis of
peptides is possible, which makes feasible the synthesis of new drugs, functional protein fragments, and antigens for
inducing the formation of specific antibodies. Nuclear magnetic resonance (NMR) spectroscopy and x-ray
crystallography are the principal techniques for elucidating three-dimensional structure, the key determinant of function.
The exploration of proteins by this array of physical and chemical techniques has greatly enriched our understanding of
the molecular basis of life and makes it possible to tackle some of the most challenging questions of biology in
molecular terms.
4.0.1. The Proteome Is the Functional Representation of the Genome
Many organisms are yielding their DNA base sequences to gene sequencers, including several metazoans. The
roundworm Caenorhabditis elegans has a genome of 97 million bases and about 19,000 protein-encoding genes, whereas
that of the fruit fly Drosophilia melanogaster contains 180 million bases and about 14,000 genes. The incredible
progress being made in gene sequencing has already culminated in the elucidation of the complete sequence of the
human genome, all 3 billion bases with an estimated 40,000 genes. But this genomic knowledge is analogous to a list of
parts for a car
it does not explain how the parts work together. A new word has been coined, the proteome, to signify a
more complex level of information content, the level of functional information, which encompasses the type, functions,
and interactions of proteins that yield a functional unit.
The term proteome is derived from proteins expressed by the genome. Whereas the genome tells us what is possible, the
proteome tells us what is functionally present
for example, which proteins interact to form a signal-transduction
pathway or an ion channel in a membrane. The proteome is not a fixed characteristic of the cell. Rather, because it
represents the functional expression of information, it varies with cell type, developmental stage, and environmental
conditions, such as the presence of hormones. The proteome is much larger than the genome because of such factors as
alternatively spliced RNA, the posttranslational modification of proteins, the temporal regulation of protein synthesis,
and varying protein-protein interactions. Unlike the genome, the proteome is not static.