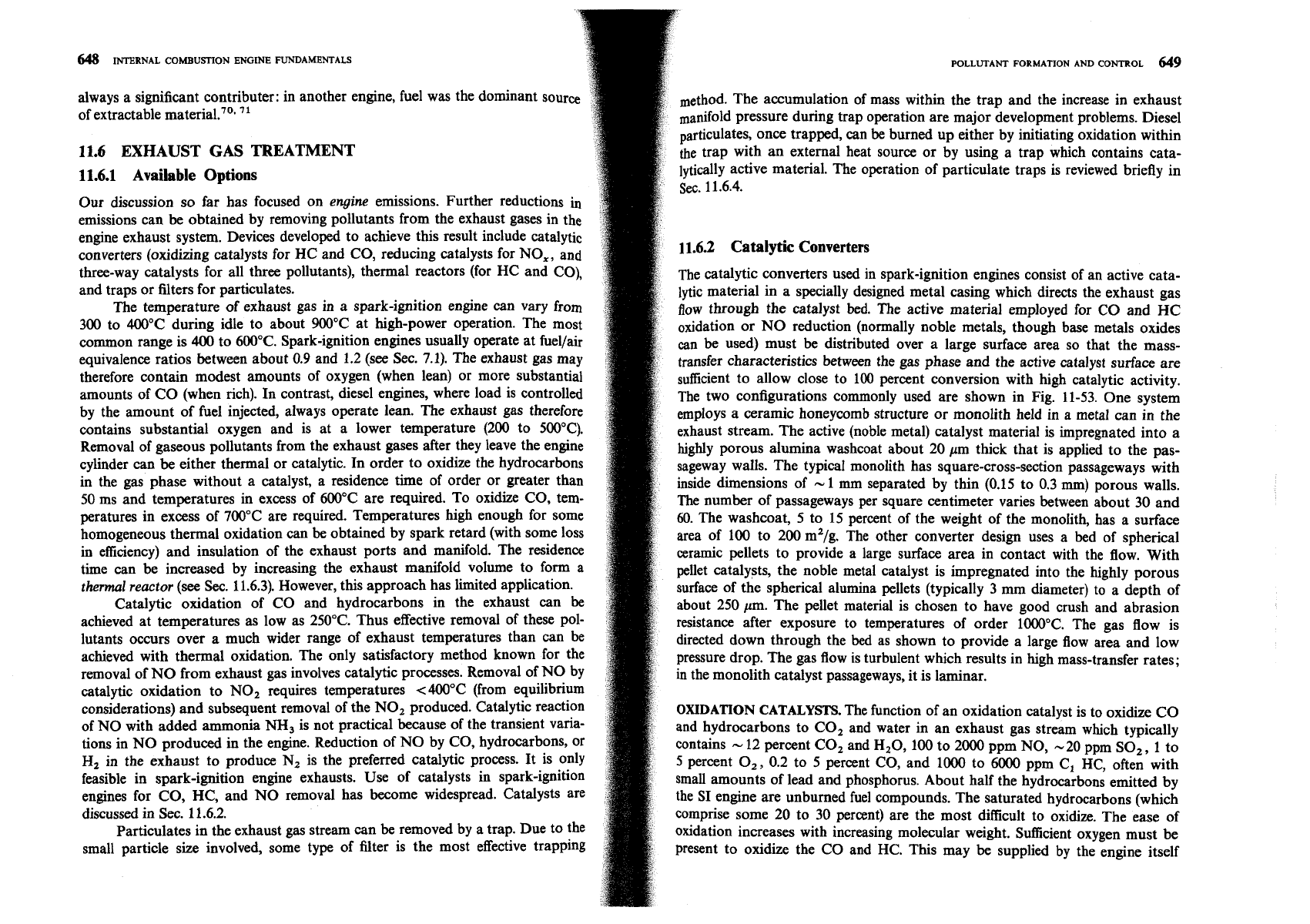
POLLUTANT FORMATION AND
(
always a significant contributer: in another engine,
of extractable material.'O*
"
11.6
EXHAUST
GAS
TREATMENT
fuel
was the dominant soul
11.6.1 Available Options
Our discussion so far has focused on
engine
emissions. Further reductions
in
emissions can be obtained by removing pollutants from the exhaust gases in the
engine exhaust system. Devices developed to achieve this result include catalytic
converters (oxidizing catalysts for HC and CO, reducing catalysts for NO,, and
three-way catalysts for all three pollutants), thermal reactors (for HC and CO),
and traps or filters for particulates.
The temperature of exhaust gas in a spark-ignition engine can vary from
300 to 400OC during idle to about 900•‹C at high-power operation. The most
common range is
400
to 6WC. Spark-ignition engines usually operate at fuellair
equivalence ratios between about 0.9 and 1.2 (see Sec. 7.1). The exhaust gas may
therefore contain modest amounts of oxygen (when lean) or more substantial
amounts of CO (when rich). In contrast, diesel engines, where load is controlled
by the amount of fuel injected, always operate lean. The exhaust gas therefore
contains substantial oxygen and is at a lower temperature (200 to 500•‹C).
Removal of gaseous pollutants from the exhaust gases after they leave the engine
cylinder can be either thermal or catalytic. In order to oxidize the hydrocarbons
in the gas phase without a catalyst, a residence time of order or greater than
50 ms and temperatures in excess of 600•‹C are required. To oxidize CO, tem-
peratures in excess of 700•‹C are required. Temperatures high enough for some
homogeneous thermal oxidation can
be
obtained by spark retard (with some loss
in efficiency) and insulation of the exhaust ports and manifold. The residence
time can be increased by increasing the exhaust manifold volume to form a
thermal reactor
(see
Sec.
11.6.3). However, this approach has limited application.
Catalytic oxidation of CO and hydrocarbons in the exhaust can be
achieved at temperatures as low as 250•‹C. Thus effective removal of these pol-
lutants occurs over a much wider range of exhaust temperatures than can be
achieved with thermal oxidation. The only satisfactory method known for the
removal of NO from exhaust gas involves catalytic processes. Removal of NO
by
catalytic oxidation to NO, requires temperatures <400"C (from equilibrium
considerations) and subsequent removal of the NO, produced. Catalytic reaction
of NO with added ammonia
NH,
is not practical because of the transient varia-
tions in NO produced in the engine. Reduction of NO by CO, hydrocarbons, or
H,
in the exhaust to produce N2 is the preferred catalytic process. It is only
feasible in spark-ignition engine exhausts. Use of catalysts in spark-ignition
engines for CO, HC, and NO removal has become widespread. Catalysts are
discussed in
Sec. 11.6.2.
Particulates in the exhaust gas stream can be removed by a trap. Due to the
small particle size involved, some type of filter is the most effective trapping
method. The accumulation of mass within the trap and the increase in exhaust
manifold pressure during trap operation are major development problems. Diesel
particulates, once trapped, can be burned up either by initiating oxidation within
the trap with an external heat source or by using a trap which contains cata-
lytically active material. The operation of particulate traps is reviewed briefly in
Sec. 11.6.4.
11.6.2 Catalytic Converters
The catalytic converters used in spark-ignition engines consist of an active cata-
lytic material in a specially designed metal casing which directs the exhaust gas
flow through the catalyst bed. The active material employed for CO and
HC
oxidation or NO reduction (normally noble metals, though base metals oxides
can be used) must be distributed over a large surface area so that the mass-
transfer characteristics between the gas phase and the active catalyst surface are
sufficient to allow close to
100 percent conversion with high catalytic activity.
The two configurations commonly used are shown in Fig. 11-53. One system
employs a ceramic honeycomb structure or monolith held in a metal can in the
exhaust stream. The active (noble metal) catalyst material is impregnated into a
highly porous alumina
washcoat about 20 pm thick that is applied to the pas-
sageway walls. The typical monolith has square-cross-section passageways with
inside dimensions of
-
1 mm separated by thin (0.15 to 0.3
mm)
porous walls.
The number of passageways per square centimeter varies between about 30 and
60.
The washcoat, 5 to 15 percent of the weight of the monolith, has a surface
area of 100 to 200 m2/g. The other converter design uses a bed of spherical
ceramic pellets to provide a large surface area in contact with the flow. With
pellet catalysts, the noble metal catalyst is impregnated into the highly porous
surface of the spherical alumina pellets (typically 3 mm diameter) to a depth of
about 250
pm. The pellet material is chosen to have good crush and abrasion
resistance after exposure to temperatures of order
1000•‹C. The gas flow is
directed down through the bed as shown to provide a large flow area and low
pressure drop. The gas flow is turbulent which results in high mass-transfer rates;
in the monolith catalyst passageways, it is laminar.
OXIDATION
CATALYSTS.
The function of an oxidation catalyst is to oxidize CO
and hydrocarbons to CO, and water in an exhaust gas stream which typically
contains
-
12 percent CO, and H,O, 100 to 2000 ppm NO, -20 ppm SO,, 1 to
5
percent 02, 0.2 to 5 percent CO, and 1000 to
6000
ppm C, HC, often with
small amounts of lead and phosphorus. About half the hydrocarbons emitted by
the SI engine are unburned fuel compounds. The saturated hydrocarbons (which
comprise some 20 to 30 percent) are the most difficult to oxidize. The ease of
oxidation increases with increasing molecular weight. Sufficient oxygen must be
present to oxidize the CO and HC. This may be supplied by the engine itself