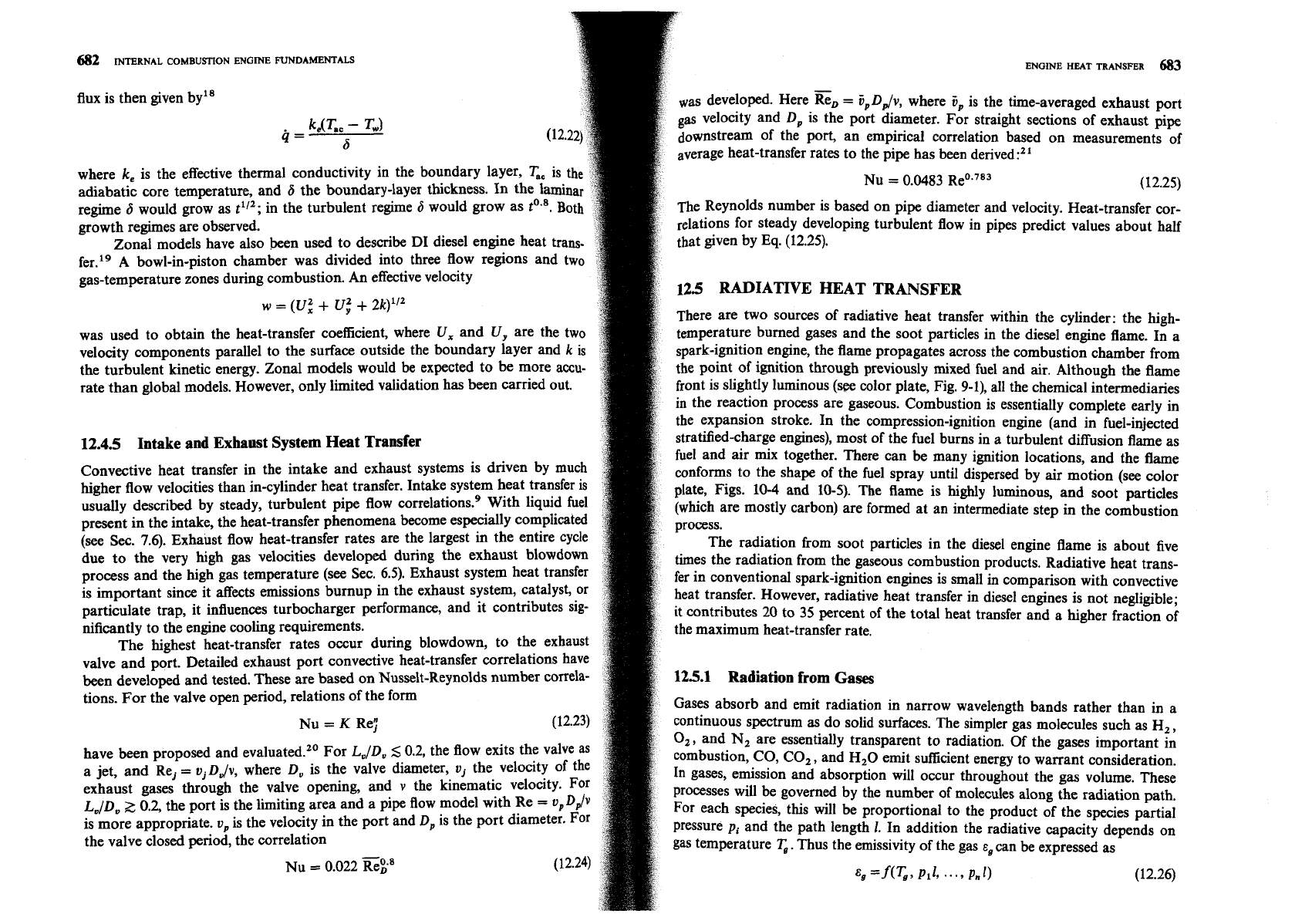
682
INTERNAL COMBUSITON ENGINE FUNDAMENTALS
ENGINE
HEAT
TRANSFER
683
flux is then given byl8
was developed. Here
ED
=
ipDdv,
where
iip
is the time-averaged exhaust port
kdT,
-
Tw)
gas velocity and
D,
is the port diameter. For straight sections of exhaust pipe
q=
6
downstream of the port, an empirical correlation based on measurements of
average heat-transfer rates to the pipe has been deri~ed:~'
where
k,
is the effective thermal conductivity in the boundary layer,
T,,
is the
Nu
=
0.0483
adiabatic core temperature, and
6
the boundary-layer thickness. In the laminar
(12.25)
regime
6
would grow as t1I2; in the turbulent regime
6
would grow as to.'. Both
The ~eynolds number is based on pipe diameter and velocity. Heat-transfer cor-
growth regimes are observed.
relations for steady developing turbulent flow in pipes predict values about half
Zonal models have also been used to describe DI diesel engine heat trans-
that given by Eq. (12.25).
fer.lg A bowl-in-piston chamber was divided into three flow regions and two
gas-temperature zones during combustion. An effective velocity
12.5
RADIATIVE HEAT TRANSFER
w
=
(U2
+
U3
+
2k)'lZ
There are two sources of radiative heat transfer within the cylinder: the high-
was used to obtain the heat-transfer coefficient, where
U,
and
U,
are the two
temperature burned gases and the soot particles in the diesel engine flame. In a
velocity components parallel to the surface outside the boundary layer and
k
is
spark-ignition engine, the flame propagates across the combustion chamber from
the turbulent kinetic energy. Zonal models would be expected to be more accu-
the point of ignition through previously mixed fuel and air. Although the flame
rate than global models. However, only limited validation has been carried out. front is slightly luminous
(see
color plate, Fig.
9-l),
all the chemical intermediaries
in the reaction process are gaseous. Combustion is essentially complete early in
the expansion stroke. In the compression-ignition engine (and in fuel-injected
12.45
Intake and Exhaust System
Heat
~ransfer
stratified-charge engines), most of the fuel burns in a turbulent diffusion flame as
fuel and air mix together. There can be many ignition locations, and the flame
Convective heat transfer in the intake and exhaust systems is driven by much
conforms to the shape of the fuel spray until dispersed by air motion (see color
higher flow velocities than in-cylinder heat transfer. Intake system heat transfer is
plate, Figs. 10-4 and
10-5). The flame is highly luminous, and soot particles
usually described by steady, turbulent pipe flow
correlation^.^
With liquid fuel
(which are mostly carbon) are formed at an intermediate step in the combustion
present in the intake, the heat-transfer phenomena become especially complicated
(see
Sec. 7.6). Exhaust flow heat-transfer rates are the largest
in
the entire cycle
The radiation from soot particles in the diesel engine flame is about five
due to the very high gas velocities developed during the exhaust blowdown
times the radiation from the gaseous combustion products. Radiative heat trans-
process and the high gas temperature
(see
Sec. 6.5). Exhaust system heat transfer
fer in conventional spark-ignition engines is small in comparison with convective
is important since it affects emissions burnup in the exhaust system, catalyst, or
heat transfer. However, radiative heat transfer in diesel engines is not negligible;
particulate trap, it influences turbocharger performance, and it contributes sig-
it contributes 20 to 35 percent of the total heat transfer and a higher fraction of
nificantly to the engine cooling requirements.
the maximum heat-transfer rate.
The highest heat-transfer rates occur during blowdown, to the exhaust
valve
and port. Detailed exhaust port convective heat-transfer correlations have
been developed and tested. These are based on Nusselt-Reynolds number correla-
125.1
Radiation from Gases
tions. For the valve open period, relations of the form
Gases absorb and emit radiation in narrow wavelength bands rather than in a
Nu
=
K
Re;
continuous sPectrum as do solid surfaces. The simpler gas molecules such as H,,
02,
and
N2
are essentially transparent to radiation. Of the gases important in
have been proposed and evaluated." For
LJD,
5
0.2, the flow exits the valve
as
combustion, CO, CO2, and Hz0 emit suflicient energy to warrant consideration.
a jet, and Rej
=
vJDJv,
where
D,
is the valve diameter,
0,
the velocity of the
In gases, emission and absorption will occur throughout the gas volume. These
exhaust gases through the valve opening, and
v
the kinematic velocity. For
Processes will be governed by the number of molecules along the radiation path.
LJD,
2
0.2, the port is the limiting area and a pipe flow model with Re
=
vp
Ddv
For each species, this will be proportional to the product of the species partial
is more appropriate.
v,
is the velocity in the port and
D,
is the port diameter. For
Pressure p, and the path length
1.
In addition the radiative capacity depends on
the valve closed period, the correlation
gas te~~erature
q
.
T'hus the emissivity of the gas
8,
can be expressed as
Nu
=
0.022
&e=f(q3
PIL
...,
P,I)
(12.26)