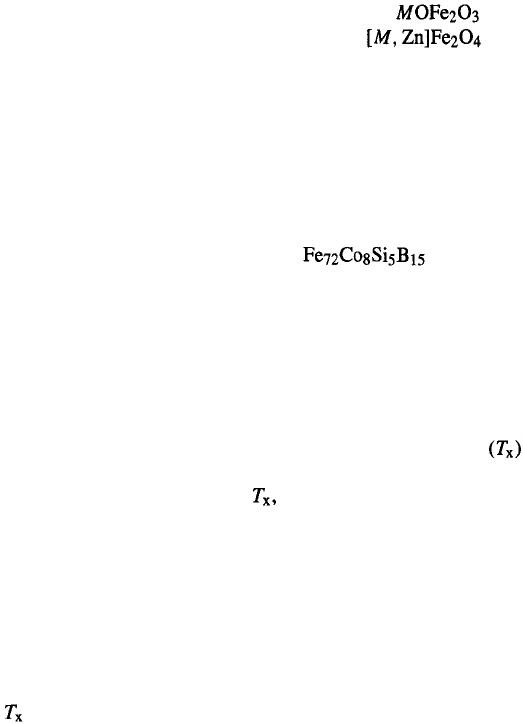
153
SECTION 14.2.
SURVEY OF MATERIALS
Soft ferrites. In contradistinction to the hard ferrites discussed in
Section 12.7, there
exists a group of ferrites that have very low magnetic anisotropy. These materials can be
visualized as consisting of mixed oxides and have the general formula with
M = Ni, Cu, or Zn. Another group can be described by the formula with
M = Cu, Mn, Ni, or Mg. Of particular interest are the ferrites composed of Mn–Zn,
Cu–Zn, Cu–Mn, Ni–Zn, Mg–Zn, and Mg–Mn. These materials are primarily used in high–
frequency applications where reduction of the various losses accompanying high-frequency
magnetization is more important than the static magnetic characteristics. These include head
applications to be discussed in a separate section below. A survey of this interesting class
of materials has been given by Brabers (1995).
Amorphous alloys. Several types of amorphous alloys have been found to exhibit soft-
magnetic properties much superior to those found in crystalline materials. For instance, the
core losses measured in amorphous alloys of the composition
have values
that are about an order of magnitude smaller than those of commercial Fe–Si sheets. Most
amorphous alloys are prepared by ejecting a molten alloy onto a rotating copper wheel (melt
spinning). The high cooling rate associated with this method suppresses crystallization.
Amorphous alloys prepared in this manner are also called metallic glasses.
In the amorphous state, the constituting atoms have a more or less random arrange-
ment, grain boundaries being absent. The amorphous state is less stable than the crystalline
amorphous-to-crystalline transformation takes place at the crystallization temperature
which depends on the composition of the alloy. Most amorphous alloys show a slight atomic
rearrangement already at temperatures somewhat below
state and this causes amorphous alloys to spontaneously crystallize upon heating. This
known as structural relaxation.
As with many crystalline soft-magnetic alloys, after casting or mechanical deformation,
a mild thermal treatment is required to remove mechanical stress that can act as a source
of coercivity. In amorphous alloys, this stress-release treatment generally does not have
the desired result because of the occurrence of structural relaxation. The reason for this
is the following. As in crystalline materials, the magnetization processes are governed by
nucleation and growth of magnetic domains. This implies that in the remanent state and in
the absence of an external magnetic field there will be a distribution of magnetic domains
and a corresponding distribution of local magnetization directions. When an amorphous
alloy is annealed (below
) under these circumstances, structural relaxation will usually
be accompanied by an increase in coercivity. This may be illustrated by means of the results
shown in Fig. 14.2.6 where the annealed material (curve B) has a substantially higher
coercivity than the original melt-spun material (curve A). This increase is a consequence
of the presence of magnetic domains with different local magnetization directions, which
causes the local structural rearrangements to proceed in a different way.
It is shown in Fig. 14.2.7 how different local-field orientations may lead to different
local rearrangements. In the schematic representation in Fig. 14.2.7, it is assumed that
pair ordering of the larger type of atoms leads to lower magnetic energy when the axis
of the pair of atoms is perpendicular to the local field. The directional ordering in each
magnetic domain therefore results in the formation of a local anisotropy. The consequence
of this is that the distribution of domains and domain walls during annealing, in the absence
of an external field becomes further stabilized and fixed at the original position. These
stabilized domain walls cause the mentioned increase in coercivity. In order to be able to
stress anneal amorphous alloys under suppression of the undesirable domain-wall fixing