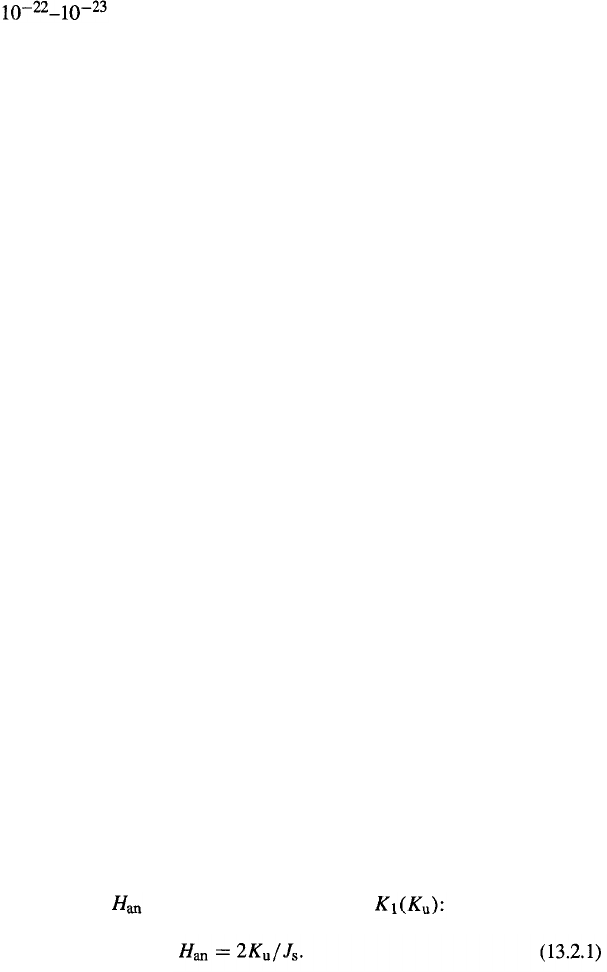
134
CHAPTER 13. HIGH-DENSITY RECORDING MATERIALS
Gambino et al. (1973) is the most prominent one and will be discussed briefly.
Gambino and
co-workers conclude that short-range ordering of the atoms is the main source of anisotropy
in sputtered Gd–Co films. These authors also provide a clue as to which
type of short-range
ordering causes the anisotropy. On the basis of studies on hep-cobalt, they conclude that the
easy magnetization direction most likely is due to the presence of Co–Co atom pairs having
their pair axes perpendicular to this direction, the magnitude of the anisotropy energy being
of the order of
J per pair.
In order to understand the formation of such pair-atoms during vapor deposition, one has
to consider the following. During the deposition process the ad-atom impinges on the film
surface with considerable energy. After impingement, it rapidly loses this energy to the
substrate and the main body of the film. If the substrate temperature is sufficiently high, the
ad-atom will be able to move by means of surface diffusion to favorable sites of relatively
low energy, so as to produce eventually a crystalline film. Low substrate temperatures and
high evaporation rates do not favor such rearrangements of the ad-atoms and then may lead
to amorphous films.
In the intermediate case, the ad-atom may still have the
opportunity to jump to any of its
nearest-neighbor surface sites, the jump probability being proportional to the corresponding
activation energy. Differences in activation energy for jumps between the initial site and the
nearest-neighbor surface site can have chemical, geometrical, and magnetic origins. This
difference in activation energy for atomic jumps can be exploited for the generation of a
higher concentration of Co pairs with their axes in the film plane than would correspond
to a statistical distribution. Use is made of so-called bias sputtering, leading to conditions
where an ad-atom bonded to a similar surface atom has a higher resputtering probability
than an ad-atom bonded to a dissimilar atom. Consequently, there will be a greater statistical
probability of Gd–Co pairs with their pair axes oriented perpendicular to the film plane than
parallel to the film plane. The opposite holds for Co–Co pairs. This behavior of Gd–Co alloys
is due to the fact that the bonding between a Co atom and a Gd atom is stronger than between
two Co atoms or two Gd atoms. This is intimately related to the negative heats of solution
of Gd in Co and of Co in Gd (see, for instance, de Boer et al., 1988). The corresponding
heat-of-solution values are by far less negative in the case of Gd–Fe. The weaker bonding
between Fe and Gd atoms is probably the reason why the perpendicular anisotropy is less
easily attained by means of this method in the Gd–Fe alloys than in the Gd–Co alloys.
There are several observations that support the pair-ordering model of anisotropy. First,
the anisotropy is relatively temperature independent near room temperature. The magnetic
ordering of the Co sublattice is almost complete at room temperature, in contrast to the Gd
sublattice that becomes magnetically ordered more gradually at lower temperatures. This
indicates that the anisotropy is to be associated with the Co sublattice. Second, the growth-
induced anisotropy increases with increasing resputtering but decreases at high deposition
rates and low substrate temperatures.
Other models dealing with the occurrence of positive uniaxial anisotropy in amor-
phous R-3d alloys consider various types of shape anisotropy associated with structural
inhomogeneities on a microstructural scale, including phase separation.
It was shown in Chapter 11 that in uniaxial materials, the following relation exists
between the anisotropy field and the anisotropy constant