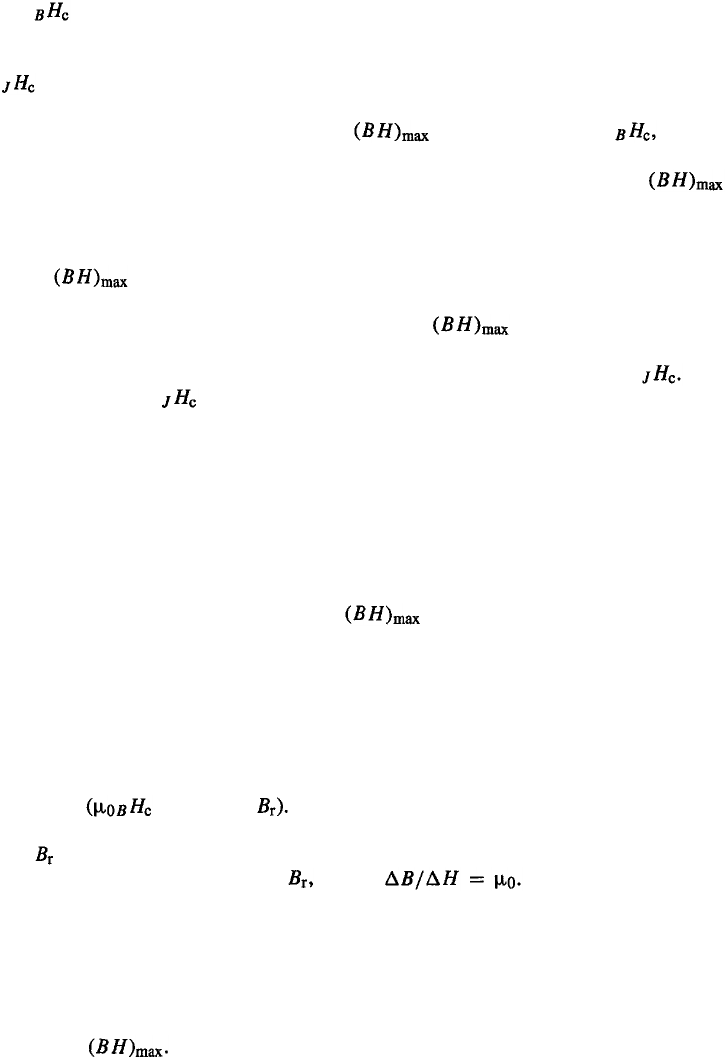
107
SECTION 12.2. SUITABILITY CRITERIA
and will not be much different from each other when the former value is smaller than
the remanence of the permanent-magnet material, as for the material B in Fig. 12.1.1.
In permanent-magnet materials based on rare-earth compounds, the intrinsic coercivity
can become much larger than the remanence. This situation is illustrated by the example
of material A shown in Fig. 12.1.1. In this case, the value of the intrinsic coercivity consid-
erably exceeds the field corresponding to the
point and also exceeds the field
where the magnetic flux vanishes. In the following, it will be assumed that both magnets
form part of a magnetic circuit and that they have a shape corresponding to their
point. When incorporated into a magnetic circuit, in which external magnetic fields are
present, the magnet material B in Fig. 12.1.1 is able to resist only a relatively small demag-
netizing field. For instance, magnetizing fields higher than twice the field corresponding
to the
point will completely demagnetize the magnet body and hence make it
useless. In contrast, the magnet material A in Fig. 12.1.1 is able to resist demagnetizing
fields more than three tunes higher than the field at its point. It may be seen from
the figure that this behavior originates from the independence of the magnetic polarization
J
(broken line) on opposite external and/or internal fields up to a value close to
High values of can generally be obtained in magnet materials that have a high
intrinsic magnetocrystalline anisotropy, as in rare-earth compounds. In materials where the
hard-magnetic properties originate from shape anisotropy (Alnico-type materials, as will
be discussed in more detail in Section 12.8), it is not possible to generate large coercivities.
The B(H) curves of representative rare-earth-based magnets are compared in Fig. 12.2.1
with the B(H) curve of Ticonal XX (Alnico type) and with the B(H) curves of some other
common types of magnet materials. It is the presence of large coercivities in particular that
makes the rare-earth-based magnets suitable for applications in which flat magnet shapes
are required.
It follows from the foregoing that the value itself is not always a sufficient
criterion for the suitability of a given permanent-magnet material to be applicable in electric
motors. More relevant to this case is the extent to which reverse fields can be applied that
leave the magnetic properties of the magnet body unchanged after removal of these fields.
The recoil line and the recoil energy are suitability criteria commonly used to characterize
permanent-magnet materials for use in permanent-magnet devices in which substantial
changes of the demagnetizing field occur in the air gap. For defining these quantities,
one may consider a magnet body characterized by a B(H) loop like the one shown in
Fig. 12.2.2
smaller than After application of a demagnetizing field up to a value
corresponding to point a, the material will generally not return along the line connecting a
and but along the line abc. This so-called recoil line has a slope similar to that of B(H)
in the first quadrant of the loop at
that is, The hatched area in the
figure (b is midway in between a and c) is commonly referred to as the recoil energy. This
energy generally depends on the location of a, meaning that there is a
maximum attainable
value for each material. A relatively high value of the maximum recoil product is reached
in magnet materials in which the high coercivity originates from a large magnetocrystalline
anisotropy and where the recoil line coincides with the B(H) curve over an extended field
range. In magnets based on shape anisotropy, the maximum recoil energy is only a small
fraction of
Magnetic devices in which cyclic operations are involved and where reversibility plays
a prominent role require quite a different criterion for the suitability ofmagnet materials. The