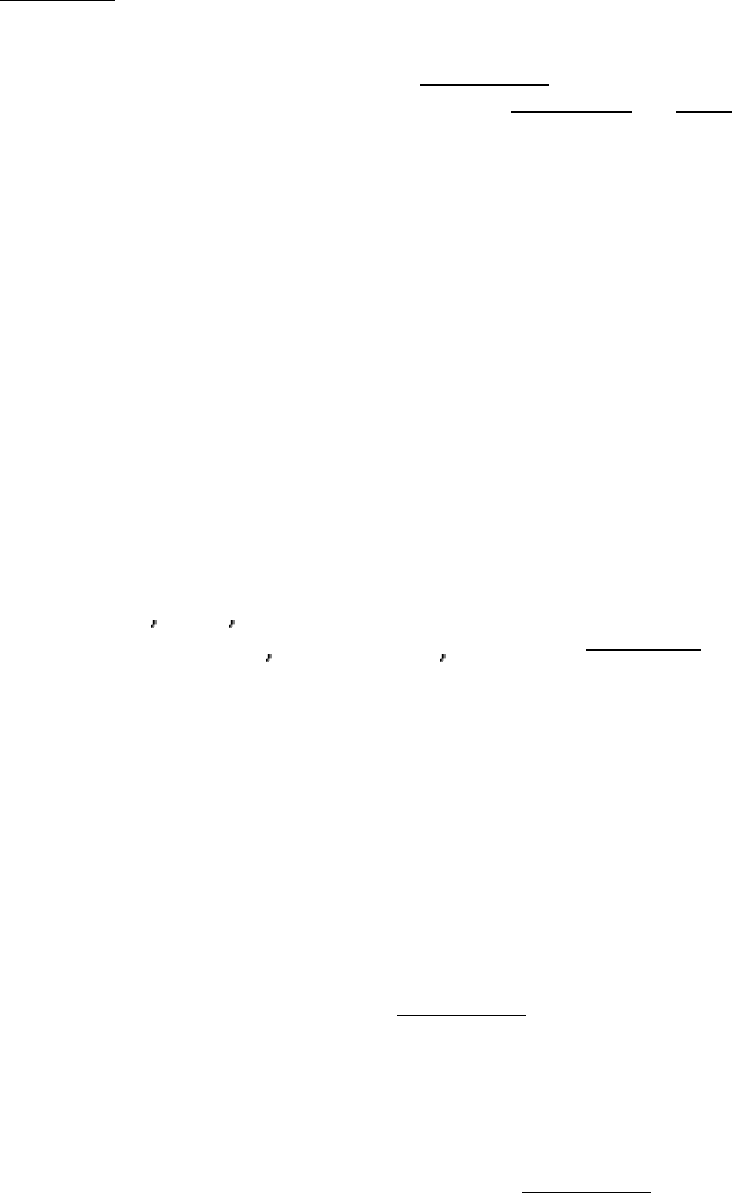
conserved from yeast through human beings.
The winding of DNA around the nucleosome core contributes to DNA's packing by decreasing its linear extent. An
extended 200-bp stretch of DNA would have a length of about 680 Å. Wrapping this DNA around the histone octamer
reduces the length to approximately 100 Å along the long dimension of the nucleosome. Thus the DNA is compacted by
a factor of seven. However, human chromosomes in metaphase, which are highly condensed, are compacted by a factor
of 10
4
. Clearly, the nucleosome is just the first step in DNA compaction. What is the next step? The nucleosomes
themselves are arranged in a helical array approximately 360 Å across, forming a series of stacked layers approximately
110 Å apart (Figure 31.18). The folding of these fibers of nucleosomes into loops further compacts DNA.
The writhing of DNA around the histone core in a left-handed helical manner also stores negative supercoils; if the DNA
in a nucleosome is straightened out, it will be underwound (Section 23.3.2). This underwinding is exactly what is needed
to separate the two DNA strands during replication and transcription (Sections 27.5 and 28.1.5).
31.2.3. The Control of Gene Expression Requires Chromatin Remodeling
Does chromatin structure play a role in the control of gene expression? Early observations suggested that it does indeed.
The treatment of cell nuclei with the nonspecific DNA-cleaving enzyme DNAse I revealed that regions adjacent to genes
that are being actively transcribed are more sensitive to cleavage than are other sites in the genome, suggesting that the
DNA in these regions is less compacted than it is elsewhere in the genome and more accessible to proteins. In addition,
some sites, usually within 1 kb of the start site of an active gene, are exquisitely sensitive to DNAse I and other
nucleases. These hypersensitive sites correspond to regions that have few nucleosomes or have nucleosomes in an altered
conformational state. Hyper-sensitive sites are cell-type specific and developmentally regulated. For example, globin
genes in the precursors of erythroid cells from 20-hour-old chicken embryos are insensitive to DNAse I. However, when
hemoglobin synthesis begins at 35 hours, regions adjacent to these genes become highly susceptible to digestion. In
tissues such as the brain that produce no hemoglobin, the globin genes remain resistant to DNAse I throughout
development and into adulthood. The results of these studies suggest that a prerequisite for gene expression is a relaxing
of the chromatin structure.
Recent experiments even more clearly revealed the role of chromatin structure in regulating access to DNA binding sites.
Genes required for galactose utilization in yeast are activated by a DNA-binding protein called GAL4, which recognizes
DNA binding sites with two 5
-CGG-3 sequences separated by 11 base pairs (Figure 31.19). Approximately 4000
potential GAL4 binding sites of the form 5
-CGG(N)
11
CCG-3 are present in the yeast genome, but only 10 of them
regulate genes necessary for galactose metabolism. What fraction of the potential binding sites are actually bound by
GAL4? This question is addressed through the use of a technique called chromatin immunoprecipitation (ChIP). GAL4
is first cross-linked to the DNA to which it is bound in chromatin. The DNA is then fragmented into small pieces, and
antibodies to GAL4 are used to isolate the chromatin fragments containing GAL4. The cross-linking is reversed, and the
DNA is isolated and characterized. The results of these studies reveal that only approximately 10 of the 4000 potential
GAL4 sites are occupied by GAL4 when the cells are growing on galactose; more than 99% of the sites appear to be
blocked. Thus, whereas in prokaryotes all sites appear to be equally accessible, chromatin structure shields a large
number of the potential binding sites in eukaryotic cells. GAL4 is thereby prevented from binding to sites that are
unimportant in galactose metabolism.
These lines of evidence and others reveal that chromatin structure is altered in active genes compared with inactive ones.
How is chromatin structure modified? As we shall see in Section 31.3.4, specific covalent modifications of histone
proteins are crucial. In addition, the binding of specific proteins to DNA sequences called enhancers at specific sites in
the genome plays a role.
31.2.4. Enhancers Can Stimulate Transcription by Perturbing Chromatin Structure
We can now understand the action of enhancers, already introduced in Section 28.2.6. Recall that these DNA sequences,
although they have no promoter activity of their own, greatly increase the activities of many promoters in eukaryotes,